Astropedology
Astropedology is the study of very ancient paleosols and meteorites relevant to the origin of life and different planetary soil systems. It is a branch of soil science (pedology) concerned with soils of the distant geologic past and of other planetary bodies to understand our place in the universe.[1] A geologic definition of soil is “a material at the surface of a planetary body modified in place by physical, chemical or biological processes”.[1] Soils are sometimes defined by biological activity but can also be defined as planetary surfaces altered in place by biologic, chemical, or physical processes.[2] By this definition, the question for Martian soils and paleosols becomes, were they alive? Astropedology symposia are a new focus for scientific meetings on soil science.[3] Advancements in understanding the chemical and physical mechanisms of pedogenesis on other planetary bodies in part led the Soil Science Society of America (SSSA) in 2017 to update the definition of soil to: "The layer(s) of generally loose mineral and/or organic material that are affected by physical, chemical, and/or biological processes at or near the planetary surface and usually hold liquids, gases, and biota and support plants".[4]
Moon
The surface of the moon is covered with lunar regolith, a mixture of fine dust and rocky debris made by meteorite impacts, considered the soil of the moon.[5] Astronauts found few rock samples to pick up on mature regolith surfaces. The rocks had all been broken to fine soil by micrometeorite bombardment over the past billion years. The bulk of lunar regolith is a fine gray soil, breccia and rock fragments of the local bedrock. Continuous attacks by micrometeorites further disintegrate and melt soil particles. This melt, mixed with lithic fragments, forms irregular clusters called agglutinates.[6] Lunar exploration has found all essential minerals for growth of plants in sufficient quantities.[7] Organic matter in the form of amino acids have been detected in lunar samples from the Apollo missions, but isotopic and molecular evidence of these compounds suggests terrestrial contamination as the source[8]
Mars
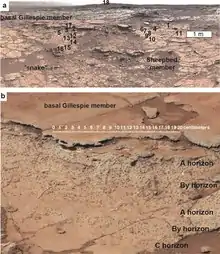
Evidence of paleosols on Mars is derived from both in-situ and orbital remote sensing investigations of the Martian surface. In-situ chemical / mineralogical analyses (Mars Science Laboratory) and images (Mastcam, MAHLI) from the Curiosity rover in Gale Crater on Mars have shown similarities with soil horizons and soil structures found on Earth.[9] Morphological evidence includes soil features such as gradational alteration and bedding disruption by dilational cracks and veins, consistent with soluans of desert soils, blocky angular ped structures, sand wedges, a shallow gypsic (By) horizon, and vesicular structure. Structures like those found in Earth's desert soils caused by microbial gas production after rains (vesicular structure) have been recognized on Mars, but definitive evidence of life on Mars has yet to be obtained. Mineral abundances within the paleosols show olivine weathering to smectite and a modest depletion of phosphorus. Such hydrolytic weathering is comparable to weathering found on Earth. The chemical and morphological features of the Yellowknife Bay paleosols are a new line of evidence for late Noachian (3.7 ± 0.3 Ga) paleoclimate on Mars, and are interpreted as forming under a hyperarid frigid paleoclimate.[9] Radiometric dating suggests the paleosols at Yellowknife Bay are 3.7 billion years old (± 0.3 billion years) and reveal a change from possible "warm and wet" conditions of the Early Noachian (~4.1-3.9 Ga) to an extreme arid and cold climate with limited soil formation.
Remote sensing of the Martian surface by the Mars Reconnaissance Orbiter (MRO)'s CRISM instrument and the Infrared Mineralogical Mapping Spectrometer (OMEGA) has detected the presence of dioctahedral and trioctahedral phyllosilicate clays in thousands of locations across the surface of the planet.[10][11][12] Orbital characterization of Martian mineralogy is primarily derived from the visible/near-infrared (VNIR) spectra of rocks containing clay minerals. These areas include Gale Crater, Mawrth Vallis, Oxia Planum, and Nili Fossae, among others, and date to 4.0-3.7 Ga.[12][13] There are two hypotheses to explain the formation and distribution of phyllosilicate clays on Mars: (1) subsurface and hydrothermal activity and/or diagenesis[14][15][16] which yield trioctahedral phyllosilicates, and (2) surface / subaerial chemical weathering, e.g., pedogenesis[10][11][12][17][18][19][20][21][22] which yield dioctahedral phyllosilicates. Importantly, some of these areas (Mawrth Vallis and Oxia Planum) have weathering profiles of Al-smectites overlain by Fe/ Mg smectites (all of which appear to be dioctahedral), and then poorly crystalline / amorphous phases like allophane and imogolite. These weathering profiles are capped by an igneous deposit of an estimated 3.7-3.6 Ga which may be a pyroclastic deposit or a mafic sandstone,[23] similar to paleosols buried beneath igneous deposits on Earth.[24][25][26] These stratigraphic profiles appear to be up to 200 m in thickness, with individual layers of 10 m in thickness or less.[23] This stratigraphy reflects the possible cooling and drying of Noachean Mars, and may preserve organic matter or other biosignatures because of the exceptionally high clay content (~50 wt %) and clay mineralogy (2:1 smectites)[27] of these buried rocks.[13]
Growing Plants on the Moon and Mars
The soil on Mars has the required nutrients plants would need to survive. Oxygen, carbon, hydrogen, nitrogen, potassium, phosphorus, calcium, magnesium, sulfur, iron, manganese, zinc, copper, molybdenum, boron, and chlorine have all been detected on Martian soil or in Martian meteorites. Depending on the exact location, fertilizers may need to be added to the soil.[28] Lunar and Mars explorations have provided information about the mineral composition of the soils on the Moon and Mars. All essential minerals for the growth of plants appear to be present in sufficient quantities in both soils probably with the exception of reactive nitrogen. Nitrogen in reactive form (NO3, NH4) is one of the essential minerals necessary for almost all plant growth. The major source of reactive nitrogen on Earth is the mineralization of organic matter. Nitrogen in reactive form (NO3, NH4) is one of the essential minerals necessary for almost all plant growth. Reactive nitrogen is part of the material in our solar system and is part of solar wind, a source of reactive nitrogen on the moon and Mars. Reactive nitrogen may also arise as an effect of lightning or volcanic activity and both processes may occur on Mars. This indicates that in principle reactive nitrogen could be present. However, the Mars Pathfinder was not able to detect reactive nitrogen. Thus the actual presence of major quantities of reactive nitrogen remains uncertain. The absence of sufficient reactive nitrogen may be solved by using nitrogen fixing species. In symbioses with bacteria these nitrogen fixers are able to bind nitrogen from the air and transform it into nitrates, a process which requires nitrogen in the atmosphere. However, there is no atmosphere on the moon, and on Mars it is only minimally present and contains traces of nitrogen. There are published reports on the first large-scale controlled experiment to investigate the possibility of growing plants in Mars and moon soil simulants. The results show that plants are able to germinate and grow on both Martian and moon soil simulant for a period of 50 days without any addition of nutrients. Growth and flowering on Mars regolith simulant was much better than on moon regolith simulant and even slightly better than on our control nutrient poor river soil. Reflexed stonecrop (a wild plant); the crops tomato, wheat, and cress; and the green manure species field mustard performed particularly well. The latter three flowered, and cress and field mustard also produced seeds. The results showed that in principle it is possible to grow crops and other plant species in Martian and Lunar soil simulants. However, many questions remain about the simulants’ water carrying capacity and other physical characteristics and also whether the simulants are representative of the real soils.[7]
Perchlorates
The presence of perchlorates in the soil makes growing vegetables on Mars especially difficult. Since there is no ozone layer on Mars, UV rays penetrate to the surface of the planet. Perchlorates become toxic when exposed to UV light, destroying bacteria within minutes of exposure. Research suggests that the iron oxides and hydrogen peroxide present in the soil on Mars increases the toxicity of perchlorates.[29] The high level of perchlorates found on Mars (0.05 wt %) is concentrated enough that it would be toxic for humans and crops, and could be used for rocket fuel.[30] Studies indicate that low concentrations of aqueous perchlorates inhibit the height, weight, chlorophyll content and oxidizing power of plants. One plant, E. crassipes, seems to have a high tolerance to perchlorates and might be an ideal plant for growing on Mars.[31] Perchlorates can accumulate in the tissue of plants if grown in a contaminated medium. Since even trace amounts interfere with thyroid functions in humans, the presence of perchlorates in Martian soils is a significant issue that needs to be addressed before colonization occurs.[32]
Early Earth
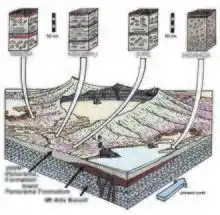
Soil profiles from the Pilbara region of northwestern Australia show distinct depletion of phosphorus. A common metabolism for sulfur bacteria is oxidation to sulphates such as gypsum and barite. These minerals are common is anaerobic acid-sulphate soils found on Earth currently and are considered a potential reason for the accumulation of sulphate in Archaean paleosols. This reflects circumstantial evidence for life in paleosols on Earth during the Archean, 3.42 to 3.46 Ga [1].
Origin of life
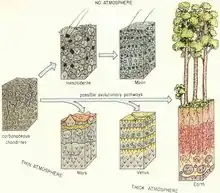
The theory that life evolved in soil is appealing because soil traps water between the grains of clay, providing an array of microscopic reaction chambers.[1] These may also promote formation of organic compounds by mechanisms demonstrated in the Urey-Miller experiment, so that planetary surfaces were covered with carbonaceous chondrite. Clayey and organic soils are protected from erosion and thus continued to produce clay and organic matter. Unicellular life, when it evolved, would also have served same purpose of binding the soil. The ingredients required for life are still provided by the weathering cycle, which promotes life.
References
- Retallack, G.J. (2016). "Astropedology: paleosols and the origin of life". Geology Today. 32 (5): 172–178. doi:10.1111/gto.12149.
- Retallack, G.J. (2001). Soils of the past. Blackwell.
- "New Frontiers of Soil and Plant Sciences: Astropedology and Space Agriculture". scisoc.confex.com. Retrieved 3 May 2019.
- van Es, Harold (5 October 2017). "A New Definition of Soil". A New Definition of Soil: SSSA.
- prohects.ncsu.edu/project/agronauts/mission3_5.htm
- curator.jsc.nasa.gov/lunar/letss/regolith.pdf
- Wamelink, G. W. Wieger; Frissel, Joep Y.; Krijnen, Wilfred H. J.; Verwoert, M. Rinie; Goedhart, Paul W.; de la Fuente, Alberto (27 August 2014). "Can Plants Grow on Mars and the Moon: A Growth Experiment on Mars and Moon Soil Simulants". PLOS ONE. 9 (8): e103138. Bibcode:2014PLoSO...9j3138W. doi:10.1371/journal.pone.0103138. PMC 4146463. PMID 25162657.
- Stiegerwald, Bill (August 6, 2017). "New NASA Study Reveals Origin of Organic Matter in Apollo Lunar Samples". nasa.gov.
- Retallack, G.J. (2014). "Paleosols and paleoenvironments of early Mars". Geology. 42 (9): 755–758. Bibcode:2014Geo....42..755R. doi:10.1130/G35912.1. S2CID 129371287.
- Bishop, J.L. et al., 2008, Phyllosilicate diversity and past aqueous activity revealed at Mawrth Vallis, Mars. Science, v. 321, 10-13, doi: 10.1126/science.1159699
- Bishop, J.L., Loizeau, D., Mckeown, N.K., Saper, L., Dyar, M.D., Des, D.J., Parente, M., and Murchie, S.L., 2013, What the ancient phyllosilicates at Mawrth Vallis can tell us about possible habitability on early Mars: Planetary and Space Science, v. 86, p. 130–149, doi: 10.1016/j.pss.2013.05.006
- Carter, J., Loizeau, D., Mangold, N., Poulet, F., and Bibring, J., 2015, Widespread sur-face weathering on early Mars : A case for a warmer and wetter climate: Icarus, v. 248, p. 373–382, doi: 10.1016/j.icarus.2014.11.011.
- Bishop, Janice; Rampe, Elizabeth B.; Velbel, Michael A.; Leslie L. Baker; Gago-Duport, Luis; Michalski, Joseph R.; Fairén, Alberto G.; Bishop, Janice L. (March 2018). "Surface clay formation during short-term warmer and wetter conditions on a largely cold ancient Mars". Nature Astronomy. 2 (3): 206–213. Bibcode:2018NatAs...2..206B. doi:10.1038/s41550-017-0377-9. ISSN 2397-3366. PMC 7008931. PMID 32042926.
- Ehlmann, Bethany L.; Mustard, John F.; Murchie, Scott L.; Bibring, Jean-Pierre; Meunier, Alain; Fraeman, Abigail A.; Langevin, Yves (2 November 2011). "Subsurface water and clay mineral formation during the early history of Mars". Nature. 479 (7371): 53–60. Bibcode:2011Natur.479...53E. doi:10.1038/nature10582. PMID 22051674. S2CID 4355955.
- McMahon, S.; Bosak, T.; Grotzinger, J. P.; Milliken, R. E.; Summons, R. E.; Daye, M.; Newman, S. A.; Fraeman, A.; Williford, K. H.; Briggs, D. E. G. (May 2018). "A Field Guide to Finding Fossils on Mars". Journal of Geophysical Research: Planets. 123 (5): 1012–1040. Bibcode:2018JGRE..123.1012M. doi:10.1029/2017JE005478. PMC 6049883. PMID 30034979.
- Michalski, Joseph R.; Cuadros, Javier; Bishop, Janice L.; Darby Dyar, M.; Dekov, Vesselin; Fiore, Saverio (October 2015). "Constraints on the crystal-chemistry of Fe/Mg-rich smectitic clays on Mars and links to global alteration trends". Earth and Planetary Science Letters. 427: 215–225. Bibcode:2015E&PSL.427..215M. doi:10.1016/j.epsl.2015.06.020.
- Michalski, J.R., Cuadros, J., Bishop, J.L., Dyar, M.D., Dekov, V., and Fiore, S., 2015, Constraints on the crystal-chemistry of Fe / Mg-rich smectitic clays on Mars and links to global alteration trends: Earth and Planetary Science Letters, v. 427, p. 215–225, doi: 10.1016/j.epsl.2015.06.020.
- Horgan, B., Baker, L., Carter, J., and Chadwick, O., 2017, Where is the climate signature in the mineral record of early Mars? Fourth Conference on Early Mars 2017, v. 3077, p. 2014–2015, doi: 10.1038/ncomms15978.
- Noe Dobrea, E.Z., McAdam, A.C., Freissinet, C., Franz, H., Belmahdi, I., Hammersley, M.R., and Stoker, C.R., 2016, Characterizing the mechanisms for the preservation of or-ganics at the Painted Desert: Lessons for MSL, Exo-Mars, and Mars 2020: 47th Lunar and Planetary Science Conference, p. Abstract #2796.
- Horgan, B., 2016, Strategies for Searching for Biosignatures in Ancient Martian Sub-Aerial Surface Environments: Biosignature Preservation and Detection in Mars Analog Environments, p. 7463, doi: 10.1089/ast.2016.1627
- Bristow, T.F., Rampe, E.B., Achilles, C.N., Blake, D.F., Chipera, S.J., Craig, P., Crisp, J.A., Marais, D.J. Des, Downs, R.T., Gellert, R., Grotzinger, J.P., Gupta, S., Hazen, R.M., Horgan, B. et al., 2018, Clay mineral diversity and abundance in sedimentary rocks of Gale crater, Mars: Science Advances, v. 4, p. 1–9.
- ] Retallack, G.J., 2014, Paleosols and paleoenvironments of early Mars: Geology, v. 42, p. 55–758, doi: 10.1130/G35912.1.
- Loizeau, Damien (2016). "Mars 2020 Candidate Landing Site Data Sheets: Mawrth Vallis" (PDF). NASA Jet Propulsion Laboratory.
- Retallack, Gregory J.; Bestland, Erick A.; Fremd, Theodore J. (1999), "Eocene and Oligocene Paleosols of Central Oregon", Special Paper 344: Eocene and Oligocene Paleosols of Central Oregon, Geological Society of America, pp. 1–192, doi:10.1130/0-8137-2344-2.1, ISBN 0813723442
- Singer, Arieh (1994). "Paleoclimate deduced from some early Jurassic basalt-derived paleosols from northern Israel". Palaeogeography, Palaeoclimatology, Palaeoecology. 111 (1–2): 73–82. Bibcode:1994PPP...111...73S. doi:10.1016/0031-0182(94)90348-4.
- Sheldon, Nathaniel (2003). "Pedogenesis and geochemical alteration of the Picture Gorge subgroup,Columbia River basalt, Oregon". GSA Bulletin. 115 (11): 1377–1387. Bibcode:2003GSAB..115.1377S. doi:10.1130/B25223.1. S2CID 15558962.
- Ehlmann, Bethany L.; Mustard, John F.; Fassett, Caleb I.; Schon, Samuel C.; Head III, James W.; Des Marais, David J.; Grant, John A.; Murchie, Scott L. (June 2008). "Clay minerals in delta deposits and organic preservation potential on Mars" (PDF). Nature Geoscience. 1 (6): 355–358. Bibcode:2008NatGe...1..355E. doi:10.1038/ngeo207. ISSN 1752-0894.
- "Can Plants Grow with Mars Soil?". 2015-10-05.
- "Toxic soil found on Mars could make growing vegies tough". 2017-07-07.
- "Toxic Mars: Astronauts Must Deal with Perchlorate on the Red Planet".
- He, Hongzhi; Gao, Haishuo; Chen, Guikui; Li, Huashou; Lin, Hai; Shu, Zhenzhen (15 May 2013). "Effects of perchlorate on growth of four wetland plants and its accumulation in plant tissues". Environmental Science and Pollution Research. 20 (10): 7301–7308. doi:10.1007/s11356-013-1744-4. PMID 23673920. S2CID 21398332.
- Hmielowski, Tracy (2017). "Extraterrestrial Soils and Space Agriculture". CSA News. 62 (5): 4–8. doi:10.2134/csa2017.62.0517.
- Retallack, G.J. (2018). "The oldest known paleosol profiles on Earth: 3.46 Ga Panorama Formation, Western Australia". Palaeogeography, Palaeoclimatology, Palaeoecology. 489: 230–248. Bibcode:2018PPP...489..230R. doi:10.1016/j.palaeo.2017.10.013.