Electroreception
Electroreception or electroception is the biological ability to perceive natural electrical stimuli.[1] It has been observed almost exclusively in aquatic or amphibious animals since water is a much better conductor than air. The known exceptions are the monotremes (echidnas and platypuses), cockroaches, and bees. Electroreception is used in electrolocation (detecting objects) and for electrocommunication.
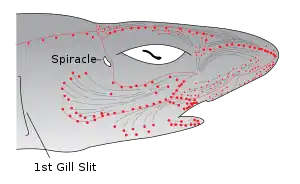
Overview
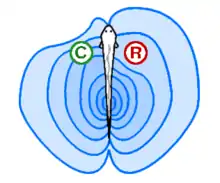
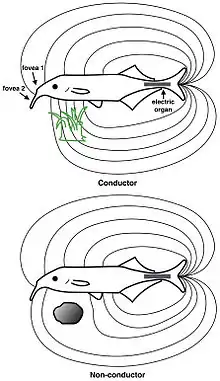
Until recently, electroreception was known only in vertebrates. Recent research has shown that bees can detect the presence and pattern of a static charge on flowers.[4] In vertebrates, electroreception is an ancestral trait, that is to say that it was present in the last common ancestor of all vertebrates.[5] This form of ancestral electroreception is called ampullary electroreception, with the receptive organs themselves called Ampullae of Lorenzini.[1] Not all vertebrates that possess electroreception have Ampullae of Lorenzini. Ampullae of Lorenzini exist in cartilaginous fishes (sharks, rays, chimaeras), lungfishes, bichirs, coelacanths, sturgeons, paddlefish, aquatic salamanders, and caecilians.[5][6] Other vertebrates that have electroreception such as catfish, gymnotiformes, Mormyridiformes, monotremes, and at least one species of cetacean all have different secondarily derived forms of electroreception.[1][7] Ampullary electroreception is passive, and is used predominantly in predation.[8][9][1] Two groups of teleost fishes are weakly electric and engage in active electroreception: the Neotropical knifefishes (Gymnotiformes) and the African elephantfishes (Notopteroidei). A rare terrestrial exception is the Western long-beaked echidna which has about 2,000 electroreceptors on its bill, compared to 40,000 for its semi-aquatic monotreme relative, the duck-billed platypus.[10]
Electrolocation
Electroreceptive animals use this sense to locate objects around them. This is important in ecological niches where the animal cannot depend on vision: for example in caves, in murky water and at night. Many fish use electric fields to detect buried prey. Some shark embryos and pups "freeze" when they detect the characteristic electric signal of their predators.[11] It has been proposed that sharks can use their acute electric sense to detect the earth's magnetic field by detecting the weak electric currents induced by their swimming or by the flow of ocean currents. The walking behaviour of cockroaches can be affected by the presence of a static electric field: they like to avoid the electric field.[12] Cabbage loopers are also known to avoid electric fields.[12]
Active electrolocation
In active electrolocation,[13] the animal senses its surrounding environment by generating electric fields and detecting distortions in these fields using electroreceptor organs. This electric field is generated by means of a specialised electric organ consisting of modified muscle or nerves. This field may be modulated so that its frequency and wave form are unique to the species and sometimes, the individual (see Jamming avoidance response). Animals that use active electroreception include the weakly electric fish, which either generate small electrical pulses (termed "pulse-type") or produce a quasi-sinusoidal discharge from the electric organ (termed "wave-type").[14] These fish create a potential which is usually smaller than one volt. Weakly electric fish can discriminate between objects with different resistance and capacitance values, which may help in identifying the object. Active electroreception typically has a range of about one body length, though objects with an electrical impedance similar to that of the surrounding water are nearly undetectable.
Passive electrolocation
In passive electrolocation, the animal senses the weak bioelectric fields generated by other animals and uses it to locate them. These electric fields are generated by all animals due to the activity of their nerves and muscles. A second source of electric fields in fish is the ion pumps associated with osmoregulation at the gill membrane. This field is modulated by the opening and closing of the mouth and gill slits.[11][15] Many fish that prey on electrogenic fish use the discharges of their prey to detect them. This behavior of a species collecting information from the communicative signals of a different species is referred to as "Eavesdropping"[16] and has been observed in the electroreceptive African sharptooth catfish (Clarias gariepinus) whilst hunting weakly electric Marcusenius macrolepidotus.[17]This has driven the prey to evolve more complex or higher frequency signals that are harder to detect.[18]
Passive electroreception is carried out solely by ampullary electroreceptors in fish. It is sensitive to low-frequency signals (from below one, and up to tens of Hertz).
Fish use passive electroreception to supplement or replace their other senses when detecting prey and predators. In sharks, sensing an electric dipole alone is sufficient to cause them to try to eat it.
Electrocommunication
Weakly electric fish can also communicate by modulating the electrical waveform they generate, an ability known as electrocommunication.[19] They may use this for mate attraction and territorial displays. Some species of catfish use their electric discharges only in agonistic displays.
In one species of Brachyhypopomus (a genus of South American river fish belonging to the family Hypopomidae, commonly known as bluntnose knifefishes), the electric discharge pattern is similar to the low voltage electrolocative discharge of the electric eel. This is hypothesised to be a form of Batesian mimicry of the powerfully-protected electric eel.[20]
Sensory mechanism
Active electroreception relies upon tuberous electroreceptors which are sensitive to high frequency (20-20,000 Hz) stimuli. These receptors have a loose plug of epithelial cells which capacitively couples the sensory receptor cells to the external environment. Passive electroreception however, relies upon ampullary receptors which are sensitive to low frequency stimuli (below 50 Hz). These receptors have a jelly-filled canal leading from the sensory receptors to the skin surface. Mormyrid electric fish from Africa use tuberous receptors known as Knollenorgans to sense electric communication signals.
Examples
Sharks and rays
Sharks and rays (members of the subclass Elasmobranchii), such as the lemon shark, rely heavily on electrolocation in the final stages of their attacks, as can be demonstrated by the robust feeding response elicited by electric fields similar to those of their prey.[21] Sharks are the most electrically sensitive animals known, responding to DC fields as low as 5 nV/cm.
The electric field sensors of sharks are called the ampullae of Lorenzini. They consist of electroreceptor cells connected to the seawater by pores on their snouts and other zones of the head. A problem with the early submarine telegraph cables was the damage caused by sharks who sensed the electric fields produced by these cables. It is possible that sharks may use Earth's magnetic field to navigate the oceans using this sense.
Bony fish
The electric eel (actually a knifefish, not an eel), besides its ability to generate high voltage electric shocks,[22] uses lower voltage pulses for navigation and prey detection in its turbid habitat.[23] This ability is shared with other gymnotiformes.
Monotremes
The monotremes are the only group of land mammals known to have evolved electroreception. While the electroreceptors in fish and amphibians evolved from mechanosensory lateral line organs, those of monotremes are based on cutaneous glands innervated by trigeminal nerves. The electroreceptors of monotremes consist of free nerve endings located in the mucous glands of the snout. Among the monotremes, the platypus (Ornithorhynchus anatinus) has the most acute electric sense.[24][25] The platypus has almost 40,000 electroreceptors arranged in a series of stripes along the bill, which probably aids the localisation of prey.[26] The platypus electroreceptive system is highly directional, with the axis of greatest sensitivity pointing outwards and downwards. By making quick head movements called "saccades" when swimming, platypuses constantly expose the most sensitive part of their bill to the stimulus to localise prey as accurately as possible. The platypus appears to use electroreception along with pressure sensors to determine the distance to prey from the delay between the arrival of electrical signals and pressure changes in the water.[25]
The electroreceptive capabilities of the two species of echidna (which are terrestrial) are much simpler. Long-beaked echidnas (genus Zaglossus) possess only 2,000 receptors and short-beaked echidnas (Tachyglossus aculeatus) have merely 400 concentrated in the tip of the snout.[26] This difference can be attributed to their habitat and feeding methods. Western long-beaked echidnas live in wet tropical forests where they feed on earthworms in damp leaf litter, so their habitat is probably favourable to the reception of electrical signals. Contrary to this is the varied but generally more arid habitat of their short-beaked relative which feeds primarily on termites and ants in nests; the humidity in these nests presumably allows electroreception to be used in hunting for buried prey, particularly after rains.[27] Experiments have shown that echidnas can be trained to respond to weak electric fields in water and moist soil. The electric sense of the echidna is hypothesised to be an evolutionary remnant from a platypus-like ancestor.[25]
Dolphins
Dolphins have evolved electroreception in structures different from those of fish, amphibians and monotremes. The hairless vibrissal crypts on the rostrum of the Guiana dolphin (Sotalia guianensis), originally associated with mammalian whiskers, are capable of electroreception as low as 4.8 μV/cm, sufficient to detect small fish. This is comparable to the sensitivity of electroreceptors in the platypus.[28] To date (June 2013), these cells have been described from only a single dolphin specimen.
Bees
Bees collect a positive static charge while flying through the air. When a bee visits a flower, the charge deposited on the flower leaks over time into the ground. Bees can detect both the presence and the pattern of electric fields on flowers, and use this information to know if a flower has been recently visited by another bee and is likely to have a reduced concentration of nectar.[4] Bees detect electric fields through insulating air by mechano-reception, not electroreception. Bees sense the electric field changes via the Johnston's organs in their antennae and possibly other mechano-receptors. They distinguish different temporal patterns and learn them. During the waggle dance, honeybees appear to use the electric field emanating from the dancing bee for distance communication.[29][30]
Effects on wildlife
It has been claimed that the electromagnetic fields generated by pylons and masts have adverse effects on wildlife; a list of 153 references to this has been published.[31]
See also
References
- Electroreception. Bullock, Theodore Holmes. New York: Springer. 2005. ISBN 978-0387231921. OCLC 77005918.CS1 maint: others (link)
- Heiligenberg, Walter (1977) Principles of Electrolocation and Jamming Avoidance in Electric Fish: A Neuroethological Approach Springer-Verlag. ISBN 9780387083674.
- Lewicki, M. S.; Olshausen, B. A.; Surlykke, A.; Moss, C. F. (2014). "Scene analysis in the natural environment". Frontiers in Psychology. 5: 199. doi:10.3389/fpsyg.2014.00199. PMC 3978336. PMID 24744740.
- Clarke, D.; Whitney, H.; Sutton, G.; Robert, D. (2013). "Detection and Learning of Floral Electric Fields by Bumblebees". Science. 340 (6128): 66–69. Bibcode:2013Sci...340...66C. doi:10.1126/science.1230883. PMID 23429701. S2CID 23742599.
- Bullock, T. H.; Bodznick, D. A.; Northcutt, R. G. (1983). "The phylogenetic distribution of electroreception: Evidence for convergent evolution of a primitive vertebrate sense modality" (PDF). Brain Research Reviews. 6 (1): 25–46. doi:10.1016/0165-0173(83)90003-6. hdl:2027.42/25137. PMID 6616267. S2CID 15603518.
- King, Benedict; Hu, Yuzhi; Long, John A. (2018-02-11). "Electroreception in early vertebrates: survey, evidence and new information". Palaeontology. 61 (3): 325–358. doi:10.1111/pala.12346.
- Lavoué, Sébastien; Miya, Masaki; Arnegard, Matthew E.; Sullivan, John P.; Hopkins, Carl D.; Nishida, Mutsumi (2012-05-14). "Comparable Ages for the Independent Origins of Electrogenesis in African and South American Weakly Electric Fishes". PLOS ONE. 7 (5): e36287. Bibcode:2012PLoSO...736287L. doi:10.1371/journal.pone.0036287. PMC 3351409. PMID 22606250.
- Kempster, R. M.; McCarthy, I. D.; Collin, S. P. (2012-02-07). "Phylogenetic and ecological factors influencing the number and distribution of electroreceptors in elasmobranchs". Journal of Fish Biology. 80 (5): 2055–2088. doi:10.1111/j.1095-8649.2011.03214.x. PMID 22497416.
- Gardiner, Jayne M.; Atema, Jelle; Hueter, Robert E.; Motta, Philip J. (2014-04-02). "Multisensory Integration and Behavioral Plasticity in Sharks from Different Ecological Niches". PLOS ONE. 9 (4): e93036. Bibcode:2014PLoSO...993036G. doi:10.1371/journal.pone.0093036. PMC 3973673. PMID 24695492.
- "Electroreception in fish, amphibians and monotremes". Map of Life. Retrieved 26 October 2012.
- Coplin, S. P.; Whitehead, D. (2004). "The functional roles of passive electroreception in non-electric fishes". Animal Biology. 54 (1): 1–25. doi:10.1163/157075604323010024.
- Jackson, C. W.; Hunt, E.; Sharkh, S.; Newland, P. L. (2011). "Static electric fields modify the locomotory behaviour of cockroaches". The Journal of Experimental Biology. 214 (Pt 12): 2020–2026. doi:10.1242/jeb.053470. PMID 21613518.
- Albert, J. S.; Crampton, W. G. (2006). "Electroreception and Electrogenesis". In Lutz, P. L. (ed.). The Physiology of Fishes. Boca Raton, FL: CRC Press. pp. 429–470. ISBN 9780849320224.
- Babineau, D.; Longtin, A.; Lewis, J. E. (2006). "Modeling the Electric Field of Weakly Electric Fish". Journal of Experimental Biology. 209 (Pt 18): 3636–3651. doi:10.1242/jeb.02403. PMID 16943504.
- Bodznick, D.; Montgomery, J. C.; Bradley, D. J. (1992). "Suppression of Common Mode Signals Within the Electrosensory System of the Little Skate Raja erinacea" (PDF). Journal of Experimental Biology. 171 (Pt 1): 107–125.
- Falk, Jay J.; ter Hofstede, Hannah M.; Jones, Patricia L.; Dixon, Marjorie M.; Faure, Paul A.; Kalko, Elisabeth K. V.; Page, Rachel A. (2015-06-07). "Sensory-based niche partitioning in a multiple predator–multiple prey community". Proceedings of the Royal Society B: Biological Sciences. 282 (1808). doi:10.1098/rspb.2015.0520. ISSN 0962-8452. PMC 4455811. PMID 25994677.
- Merron, G. S. (1993). "Pack-hunting in two species of catfish, Clavias gariepinus and C. ngamensis, in the Okavango Delta, Botswana". Journal of Fish Biology. 43 (4): 575–584. doi:10.1111/j.1095-8649.1993.tb00440.x. ISSN 1095-8649.
- Stoddard, P. K. (2002). "The evolutionary origins of electric signal complexity". Journal of Physiology - Paris. 96 (5–6): 485–491. doi:10.1016/S0928-4257(03)00004-4. PMID 14692496. S2CID 6240530.
- Hopkins, C. D. (1999). "Design features for electric communication". Journal of Experimental Biology. 202 (Pt 10): 1217–1228. PMID 10210663.
- Stoddard, P. K. (1999). "Predation enhances complexity in the evolution of electric fish signals". Nature. 400 (6741): 254–256. Bibcode:1999Natur.400..254S. doi:10.1038/22301. PMID 10421365. S2CID 204994529.
- Fields, R. Douglas (August 2007). "The Shark's Electric Sense" (PDF). Scientific American. 297 (2): 74–80. Bibcode:2007SciAm.297b..74F. doi:10.1038/scientificamerican0807-74. PMID 17894175. Retrieved 2 December 2013.
- Catania, Kenneth C. (October 2015). "Electric Eels Concentrate Their Electric Field to Induce Involuntary Fatigue in Struggling Prey". Current Biology. 25 (22): 2889–2898. doi:10.1016/j.cub.2015.09.036. PMID 26521183.
- Froese, Rainer and Pauly, Daniel, eds. (2005). "Electrophorus electricus" in FishBase. December 2005 version.
- Scheich, H.; Langner, G.; Tidemann, C.; Coles, R. B.; Guppy, A. (1986). "Electroreception and electrolocation in platypus". Nature. 319 (6052): 401–402. Bibcode:1986Natur.319..401S. doi:10.1038/319401a0. PMID 3945317. S2CID 4362785.
- Pettigrew, J. D. (1999). "Electroreception in Monotremes" (PDF). The Journal of Experimental Biology. 202 (Pt 10): 1447–1454. PMID 10210685.
- "Electroreception in fish, amphibians and monotremes". Map Of Life. 2010. Retrieved June 12, 2013.
- Proske, U.; Gregory, J. E.; Iggo, A. (1998). "Sensory receptors in monotremes". Philosophical Transactions of the Royal Society B. 353 (1372): 1187–1198. doi:10.1098/rstb.1998.0275. PMC 1692308. PMID 9720114.
- Czech-Damal, N. U.; Liebschner, A.; Miersch, L.; Klauer, G.; Hanke, F. D.; Marshall, C.; Dehnhardt, G.; Hanke, W. (2012). "Electroreception in the Guiana dolphin (Sotalia guianensis)". Proceedings of the Royal Society B. 279 (1729): 663–668. doi:10.1098/rspb.2011.1127. PMC 3248726. PMID 21795271.
- Greggers, U.; Koch, G.; Schmidt, V.; Dürr, A.; Floriou-Servou, A.; Piepenbrock, D.; Göpfert, M. C.; Menzel, R. (2013). "Reception and learning of electric fields". Proceedings of the Royal Society B. 280 (1759): 1471–2954. doi:10.1098/rspb.2013.0528. PMC 3619523. PMID 23536603. 20130528.
- Greggers, U. "ESF in bees". Free University Berlin.
- "Is electrosmog harming our wildlife?". EMFsafety. 2012. Retrieved July 11, 2013.
External links
- ReefQuest Centre for Shark Research
- Electrolocation on Scholarpedia
- Video clips of Gnathonemus, Apteronotus, and Ameiurus
- Electroreception in fish, amphibians and monotremes — Map of Life, University of Cambridge
![]() |
Wikimedia Commons has media related to Electroreception. |