Ff phages
The Ff phages are a group of filamentous bacteriophage (inovirus) including f1, fd and M13, which infect bacteria bearing the F fertility factor and have a filamentous morphology.[1][2][3][4][5][6][7] These phages have a non-lytic lifecycle, in which infected bacteria are not killed, but continuously release viral particles, in contrast to the lytic and lysogenic cycles of other phages where the host is killed when phage particles are released. These phages have been used to study basic aspects of molecular biology, including membrane proteins. George Smith and Greg Winter used f1 and fd for their work on phage display for which they were awarded a share of the 2018 Nobel Prize in Chemistry.
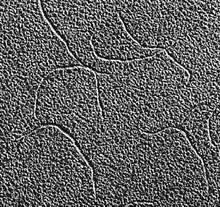
Structure
The virus particle is a flexible filament (worm-like chain) about 6 nm in diameter and 900 nm long. Several thousand copies of a small (50 amino-acid residues) elongated alpha-helical major coat protein subunit (p8) in an overlapping shingle-like array form a hollow cylinder enclosing the circular single-stranded DNA genome. Each p8 subunit has a collection of basic residues near the C-terminus of the elongated protein and acidic residues near the N-terminus; these two regions are separated by about 20 hydrophobic (non-polar) residues. The shingle-like arrangement places the basic residues near the centre of the cylinder, where they interact with the negatively-charged DNA phosphates. Longer[8] (or shorter[9]) DNA can be packaged, since more (or fewer) p8 subunits can be added during assembly as required to protect the DNA, making the phage useful for genetic studies. The acidic residues of p8 are located near the outside surface of the cylinder, where they cause the virus particle to be negatively-charged; and the non-polar regions are near non-polar regions of neighbouring p8 subunits, where non-polar interactions contribute to a notable physical stability of the virus particle. About 5 copies each of four minor proteins cap the two ends of the virus particle.[10]
_assembled_major_coat_protein%252C_exploded_view.tif.jpg.webp)
Genetics
The DNA sequence of the fd genome has 6408 nucleotide comprising 9 genes, but it produces 11 proteins, since two genes, gene 2 and gene 1, have internal in-frame translation starts, generating two additional proteins, p10 and p11. It also contains a short non-coding intergenic sequence.[11] M13 and f1 sequences are slightly different. They both have only 6407 nucleotides; f1 differs from fd in 180 positions (only 10 of these changes are reflected in amino-acid changes in gene products)[12] and M13 has only 59 nucleotide differences from f1. M13 was used in early experiments on Ff phages to identify gene functions,[13][14] and M13 was also developed as a cloning vehicle,[15] so the name "M13 phage" is often used informally as shorthand for "Ff phage". For many purposes the phages in the Ff group can be considered as interchangeable.
Five gene products are part of the virus particle: the major coat protein (p8) and the minor proteins capping the two ends, p3 and p6 at one end, and p7 and p9 at the other end. Three gene products (p2, p5, and p10) are cytoplasmic proteins needed for DNA synthesis and the rest are membrane proteins involved in assembly of the virus particle.[16]
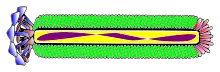
Life cycle
Infection
The p3 protein is anchored to one end of the phage particle by the C-terminal domain of p3. Infection of host bacteria involves interaction of two different N-terminal regions of p3 with two different sites of the host bacteria. First, the N2 domain of p3 attaches to the outer tip of the F-pilus, and the pilus retracts into the cell. This retraction may involve depolymerization of the pilus subunit assembly into the cell membrane at the base of the pilus by a reversal of the pilus growth and polymerization process.[1][17][18] As the tip of the pilus bearing p3 approaches the cell wall, the N1 domain of p3 interacts with the bacterial TolA protein to complete infection and release the genome into the cytoplasm of the host.[19][20]
Replication
After the single-stranded circular viral DNA enters the cytoplasm, it serves as a template for the synthesis of a complementary DNA strand. This synthesis is initiated in the intergenic region of the DNA sequence by host RNA polymerase, which synthesizes a short RNA primer on the infecting DNA as template. The host DNA polymerase III then uses this primer to synthesize the full complementary strand of DNA, yielding a double-stranded circle, sometimes called the replicative form (RF) DNA. The complementary strand of the RF is the transcription template for phage coded proteins, especially p2 and p10, which are necessary for further DNA replication.
The p2 protein cleaves the viral strand of the RF DNA, and host DNA polymerase III synthesizes a new viral strand. The old viral strand is displaced as the new one is synthesized. When a circle is complete, the covalently linked p2 cuts the displaced viral strand at the junction between the old and newly synthesized DNA and re-ligates the two ends and liberates p2. RF replicates by this rolling circle mechanism to generate dozens of copies of the RF. When the concentration of phage proteins has increased, new viral strands are coated by the replication/assembly protein p5 rather than by the complementary DNA strands, The p5 also inhibits translation of p2, so that progeny viral ssDNA production and packaging are in synchrony.[7]
Extrusion
When sufficient copies of the RF and p5 have formed, new ssDNA viral genomes get bound by p5 by interaction with a specific sequence on the DNA called the packing signal. This prevents its conversion to RF DNA. The interaction between the DNA and the p5 is weak except at the packing signal. The ssDNA-p5 complex interacts with the membrane-bound p1/p11/p4 proteins which contribute to the extrusion process, and also with the p7 and p9 proteins which form the tip of the progeny virus As the DNA is stripped of the p5, it is extruded across the membrane and wrapped in a helical casing of p8.[10]
Applications
Biological and medical sciences
These phage can be engineered to display immunogenic peptides which may be useful as vaccines.[21]
Inovirus | |
---|---|
Virus classification ![]() | |
(unranked): | Virus |
Realm: | Monodnaviria |
Kingdom: | Loebvirae |
Phylum: | Hofneiviricota |
Class: | Faserviricetes |
Order: | Tubulavirales |
Family: | Inoviridae |
Genus: | Inovirus |
See also
References
- Rasched I, Oberer E (December 1986). "Ff coliphages: structural and functional relationships". Microbiological Reviews. 50 (4): 401–27. PMC 373080. PMID 3540571.
- Mai-Prochnow A, Hui JG, Kjelleberg S, Rakonjac J, McDougald D, Rice SA (July 2015). "'Big things in small packages: the genetics of filamentous phage and effects on fitness of their host'". FEMS Microbiology Reviews. 39 (4): 465–87. doi:10.1093/femsre/fuu007. PMID 25670735.
- Rakonjac J, Bas B, Derda R, eds. (2017). Filamentous Bacteriophage in Bio/Nano/Technology, Bacterial Pathogenesis and Ecology. Frontiers Research Topics. Frontiers Media SA. doi:10.3389/978-2-88945-095-4. ISBN 978-2-88945-095-4.
- Roux S, Krupovic M, Daly RA, Borges AL, Nayfach S, Schulz F, et al. (November 2019). "Cryptic inoviruses revealed as pervasive in bacteria and archaea across Earth's biomes". Nature Microbiology. 4 (11): 1895–1906. doi:10.1038/s41564-019-0510-x. PMC 6813254. PMID 31332386.
- Morag O, Abramov G, Goldbourt A (December 2011). "Similarities and differences within members of the Ff family of filamentous bacteriophage viruses". The Journal of Physical Chemistry. B. 115 (51): 15370–9. doi:10.1021/jp2079742. PMID 22085310.
- Hay ID, Lithgow T (June 2019). "Filamentous phages: masters of a microbial sharing economy". EMBO Reports. 20 (6). doi:10.15252/embr.201847427. PMC 6549030. PMID 30952693.
- Rakonjac J, Bennett NJ, Spagnuolo J, Gagic D, Russel M (2011). "Filamentous bacteriophage: biology, phage display and nanotechnology applications". Current Issues in Molecular Biology. 13 (2): 51–76. PMID 21502666.
- Herrmann R, Neugebauer K, Zentgraf H, Schaller H (February 1978). "Transposition of a DNA sequence determining kanamycin resistance into the single-stranded genome of bacteriophage fd". Molecular & General Genetics. 159 (2): 171–8. doi:10.1007/BF00270890. PMID 345091.
- Sattar S, Bennett NJ, Wen WX, Guthrie JM, Blackwell LF, Conway JF, Rakonjac J (2015). "Ff-nano, short functionalized nanorods derived from Ff (f1, fd, or M13) filamentous bacteriophage". Frontiers in Microbiology. 6: 316. doi:10.3389/fmicb.2015.00316. PMC 4403547. PMID 25941520.
- Straus SK, Bo HE (2018). "Filamentous Bacteriophage Proteins and Assembly". Sub-Cellular Biochemistry. 88: 261–279. doi:10.1007/978-981-10-8456-0_12. PMID 29900501.
- Beck E, Sommer R, Auerswald EA, Kurz C, Zink B, Osterburg G, et al. (December 1978). "Nucleotide sequence of bacteriophage fd DNA". Nucleic Acids Research. 5 (12): 4495–503. doi:10.1093/nar/5.12.4495. PMC 342768. PMID 745987.
- Beck E, Zink B (December 1981). "Nucleotide sequence and genome organisation of filamentous bacteriophages fl and fd". Gene. 16 (1–3): 35–58. doi:10.1016/0378-1119(81)90059-7. PMID 6282703.
- Pratt D, Tzagoloff H, Erdahl WS (November 1966). "Conditional lethal mutants of the small filamentous coliphage M13. I. Isolation, complementation, cell killing, time of cistron action". Virology. 30 (3): 397–410. doi:10.1016/0042-6822(66)90118-8. PMID 5921643.
- Pratt D, Tzagoloff H, Beaudoin J (September 1969). "Conditional lethal mutants of the small filamentous coliphage M13. II. Two genes for coat proteins". Virology. 39 (1): 42–53. doi:10.1016/0042-6822(69)90346-8. PMID 5807970.
- Messing J (April 1991). "Cloning in M13 phage or how to use biology at its best". Gene. 100: 3–12. doi:10.1016/0378-1119(91)90344-b. PMID 2055478.
- Russel M, Linderoth NA, Sali A (June 1997). "Filamentous phage assembly: variation on a protein export theme". Gene. 192 (1): 23–32. doi:10.1016/s0378-1119(96)00801-3. PMID 9224870.
- Lawley TD, Klimke WA, Gubbins MJ, Frost LS (July 2003). "F factor conjugation is a true type IV secretion system". FEMS Microbiology Letters. 224 (1): 1–15. doi:10.1016/S0378-1097(03)00430-0. PMID 12855161.
- Craig L, Forest KT, Maier B (July 2019). "Type IV pili: dynamics, biophysics and functional consequences". Nature Reviews. Microbiology. 17 (7): 429–440. doi:10.1038/s41579-019-0195-4. PMID 30988511.
- Bennett NJ, Rakonjac J (February 2006). "Unlocking of the filamentous bacteriophage virion during infection is mediated by the C domain of pIII". Journal of Molecular Biology. 356 (2): 266–73. doi:10.1016/j.jmb.2005.11.069. PMID 16373072.
- Hoffmann-Thoms S, Jakob RP, Schmid FX (April 2014). "Energetic communication between functional sites of the gene-3-protein during infection by phage fd". Journal of Molecular Biology. 426 (8): 1711–22. doi:10.1016/j.jmb.2014.01.002. PMID 24440124.
- Prisco A, De Berardinis P (2012). "Filamentous bacteriophage fd as an antigen delivery system in vaccination". International Journal of Molecular Sciences. 13 (4): 5179–94. doi:10.3390/ijms13045179. PMC 3344273. PMID 22606037.