Slow-wave sleep
Slow-wave sleep (SWS), often referred to as deep sleep, consists of stage three of non-rapid eye movement sleep.[2] Initially, SWS consisted of both Stage 3, which has 20–50 percent delta wave activity, and Stage 4, which has more than 50 percent delta wave activity.[3]
Overview
This period of sleep is called slow-wave sleep because the EEG activity is synchronized, characterised by slow waves with a frequency range of 0.5–4.5 Hz, relatively high amplitude power with peak-to-peak amplitude greater than 75µV. The first section of the wave signifies a "down state", which is an inhibition or hyperpolarizing phase in which the neurons in the neocortex are silent. This is the period when the neocortical neurons are able to rest. The second section of the wave signifies an "up state", which is an excitation or depolarizing phase in which the neurons fire briefly at a high rate. The principal characteristics during slow-wave sleep that contrast with REM sleep are moderate muscle tone, slow or absent eye movement, and lack of genital activity.[4]
Slow-wave sleep is considered important for memory consolidation.[5] This is sometimes referred to as "sleep-dependent memory processing".[6] Impaired memory consolidation has been seen in individuals with primary insomnia who thus do not perform as well as those who are healthy in memory tasks following a period of sleep.[7][8] Furthermore, slow-wave sleep improves declarative memory (which includes semantic and episodic memory). A central model has been hypothesized that the long-term memory storage is facilitated by an interaction between the hippocampal and neocortical networks.[7] In several studies, after the subjects have had training to learn a declarative memory task, the density of human sleep spindles present was significantly higher than the signals observed during the control tasks that involved similar visual stimulation and cognitively-demanding tasks but did not require learning.[9][10] This associated with the spontaneously occurring wave oscillations that account for the intracellular recordings from thalamic and cortical neurons.[11]
Specifically, SWS presents a role in spatial declarative memory. Reactivation of the hippocampus during SWS is detected after the spatial learning task.[12] In addition, a correlation can be observed between the amplitude of hippocampal activity during SWS and the improvement in spatial memory performance, such as route retrieval, on the following day.[13]
A memory reactivation experiment during SWS was conducted using odor as a cue, given that it does not disturb ongoing sleep, over a prior learning task and sleep sessions. The region of the hippocampus was activated in response to odor re-exposure during SWS. This stage of sleep has an exclusive role as a context cue that reactivates the memories and favors their consolidation.[12] A further study demonstrated that when subjects heard sounds associated with previously shown pictures-locations, the reactivation of individual memory representations was significantly higher during SWS compared to other sleep stages.[14]
Affective representations are generally better remembered during sleep compared to neutral ones. Emotions with negative salience presented as a cue during SWS show better reactivation, therefore an enhanced consolidation in comparison to neutral memories. The former was predicted by sleep spindles over SWS, which discriminates the memory processes during sleep as well as facilitating emotional memory consolidation.[14]
Acetylcholine plays an essential role in hippocampus-dependent memory consolidation. An increased level of cholinergic activity during SWS is known to be disruptive for memory processing. Considered that acetylcholine is a neurotransmitter that modulates the direction of information flow between the hippocampus and neocortex during sleep, its suppression is necessary during SWS in order to consolidate sleep-related declarative memory.[15]
Sleep deprivation studies with humans suggest that the primary function of slow-wave sleep may be to allow the brain to recover from its daily activities. Glucose metabolism in the brain increases as a result of tasks that demand mental activity.[16] Another function affected by slow-wave sleep is the secretion of growth hormone, which is always greatest during this stage.[17] It is also thought to be responsible for a decrease in sympathetic and increase in parasympathetic neural activity.[17]
Prior to 2007 the American Academy of Sleep Medicine (AASM) divided Slow-wave sleep into stages 3 and 4.[18][19][20] The two stages are now combined as "Stage three" or N3. An epoch (30 seconds of sleep) which consists of 20% or more slow-wave (delta) sleep is now considered to be stage three.
Electroencephalographic characteristics
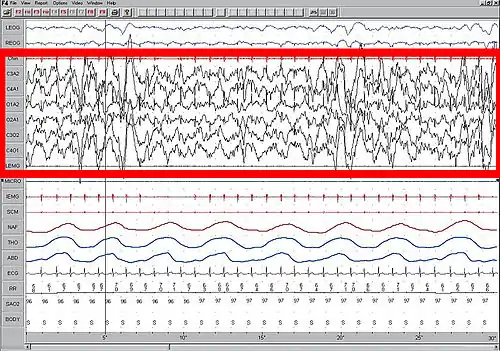
High amplitude EEG is highlighted in red.
Large 75-microvolt (0.5–2.0 Hz) delta waves predominate the electroencephalogram (EEG). Stage N3 is defined by the presence of 20% delta waves in any given 30-second epoch of the EEG during sleep, by the current 2007 AASM guidelines.[20]
Longer periods of SWS occur in the first part of the night, primarily in the first two sleep cycles (roughly three hours). Children and young adults will have more total SWS in a night than older adults. The elderly may not go into SWS at all during many nights of sleep.
Slow-wave sleep is an active phenomenon probably brought about by the activation of serotonergic neurons of the raphe system.[21]
The slow-wave seen in the cortical EEG is generated through thalamocortical communication through the thalamocortical (TC) neurons.[22] In the TC neurons this is generated by the "slow oscillation" and is dependent on membrane potential bistability, a property of these neurons due to an electrophysiological component known as "I t window". "I t window" is due to the overlap underneath activation and inactivation curves if plotted for T-type calcium channels (inward current). If these two curves are multiplied, and another line superimposed on the graph to show a small Ik leak current (outward), then the interplay between these inward (I t window) and outward (small Ik leak), three equilibrium points are seen at −90, −70 and −60 mv, −90 and −60 being stable and −70 unstable. This property allows the generation of slow waves due to an oscillation between two stable points. It is important to note that in in vitro, mGluR must be activated on these neurons to allow a small Ik leak, as seen in in vivo situations.
Functions
Hemispheric asymmetries in the human sleep
Slow-wave sleep is necessary for survival. Some animals, such as dolphins and birds, have the ability to sleep with only one hemisphere of the brain, leaving the other hemisphere awake to carry out normal functions and to remain alert. This kind of sleep is called unihemispheric slow-wave sleep and it is also partially observable in human beings. Indeed, a study reported a unilateral activation of the somatosensorial cortex when a vibrating stimulus was put on the hand of human subjects. The recordings show an important inter-hemispheric change during the first hour of non-REM sleep and consequently the presence of a local and use-dependent aspect of sleep.[23] Another experiment detected a greater number of delta waves in the frontal and central regions of the right hemisphere.[24]
Considering that SWS is the only sleep stage that reports human deep sleep as well as being used in studies with mammals and birds, it is also adopted in experiments revealing the role of hemispheric asymmetries during sleep. A predominance of the left hemisphere in the neural activity can be observed in the default-mode network during SWS. This asymmetry is correlated with the sleep-onset-latency, which is a sensitive parameter of the so-called first night effect, in other words of the reduced quality of sleep during the first session in the laboratory.[25]
The left hemisphere is shown to be more sensitive to deviant stimuli during the first night compared to the following nights of an experiment. This asymmetry explains further the reduced sleep of half the brain during SWS. Indeed, in comparison to the right one, the left hemisphere plays a vigilant role during SWS.[25]
Furthermore, a faster behavioral reactivity is detected in the left hemisphere during SWS of the first night. The rapid awakening is correlated to the regional asymmetry in the activities of SWS. These findings show that the hemispheric asymmetry in SWS plays a role as a protective mechanism. SWS is therefore sensitive to danger and non-familiar environment, creating a need for vigilance and reactivity during sleep.[25]
Neural control of slow-wave sleep
Several neurotransmitters are involved in sleep and waking patterns: acetylcholine, norepinephrine, serotonin, histamine, and orexin.[26] Neocortical neurons fire spontaneously during slow-wave sleep, thus they seem to play a role during this period of sleep. Also, these neurons appear to have some sort of internal dialogue, which accounts for the mental activity during this state where there is no information from external signals because of the synaptic inhibition at the thalamic level. The rate of recall of dreams during this state of sleep is relatively high compared to the other levels of the sleep cycle. This indicates that mental activity is closer to real life events.[11]
Physical healing and growth
Slow-wave sleep is the constructive phase of sleep for recuperation of the mind-body system in which it rebuilds itself after each day. Substances that have been ingested into the body while an organism is awake are synthesized into complex proteins of living tissue. Growth hormones are also secreted to facilitate the healing of muscles as well as repairing damage to any tissues. Lastly, glial cells within the brain are restored with sugars to provide energy for the brain.[27]
Learning and synaptic homeostasis
Learning and memory formation occurs during wakefulness by the process of long-term potentiation; SWS is associated with the regulation of synapses thus potentiated. SWS has been found to be involved in the downscaling of synapses, in which strongly stimulated or potentiated synapses are kept while weakly potentiated synapses either diminish or are removed.[28] This may be helpful for recalibrating synapses for the next potentiation during wakefulness and for maintaining synaptic plasticity. Notably, new evidence is showing that reactivation and rescaling may be co-occurring during sleep.[29]
Problems associated with slow-wave sleep
Bedwetting, night terrors, and sleepwalking are all common behaviors that can occur during stage three of sleep. These occur most frequently amongst children, who then generally outgrow them.[16] Another problem that may arise is sleep-related eating disorder. An individual will sleep-walk leaving his or her bed in the middle of the night seeking out food, and will eat not having any memory of the event in the morning.[16] Over half of individuals with this disorder become overweight.[30] Sleep-related eating disorder can usually be treated with dopaminergic agonists, or topiramate, which is an anti-seizure medication. This nocturnal eating throughout a family suggests that heredity may be a potential cause of this disorder.[16]
Effects of sleep deprivation
J. A. Horne (1978) reviewed several experiments with humans and concluded that sleep deprivation has no effects on people's physiological stress response or ability to perform physical exercise. It did, however, have an effect on cognitive functions. Some people reported distorted perceptions or hallucinations and lack of concentration on mental tasks. Thus, the major role of sleep does not appear to be rest for the body, but rest for the brain.
When sleep-deprived humans sleep normally again, the recovery percentage for each stage of sleep is not the same. Only seven percent of stages one and two are regained, but 68 percent of stage-four slow-wave sleep and 53 percent of REM sleep are regained. This suggests that stage-four sleep (known today as the deepest part of stage-three sleep) is more important than the other stages.
During slow-wave sleep, there is a significant decline in cerebral metabolic rate and cerebral blood flow. The activity falls to about 75 percent of the normal wakefulness level. The regions of the brain that are most active when awake have the highest level of delta waves during slow-wave sleep. This indicates rest is geographical. The “shutting down” of the brain accounts for the grogginess and confusion if someone is awakened during deep sleep since it takes the cerebral cortex time to resume its normal functions.
According to J. Siegel (2005), sleep deprivation results in the build-up of free radicals and superoxides in the brain. Free radicals are oxidizing agents that have one unpaired electron, making them highly reactive. These free radicals interact with electrons of biomolecules and damage cells. In slow-wave sleep, the decreased rate of metabolism reduces the creation of oxygen byproducts, thereby allowing the existing radical species to clear. This is a means of preventing damage to the brain.[31]
Amyloid beta pathology
The accumulation of amyloid beta (Aβ) in the prefrontal cortex is associated with the disruption or reduction of slow waves of NREM sleep. Therefore, this may reduce the ability for memory consolidation in older adults.[32]
Individual differences
Though SWS is fairly consistent within the individual, it can vary across individuals. Age and gender have been noted as two of the biggest factors that affect this period of sleep. Aging is inversely proportional to the amount of SWS beginning by midlife and therefore, SWS declines with age. Sex differences have also been found, such that females tend to have higher levels of SWS compared to males, at least up until menopause. There have also been studies that have shown differences between races. The results showed that there was a lower percentage of SWS in African Americans compared to Caucasians, but since there are many influencing factors (e.g. body mass index, sleep-disordered breathing, obesity, diabetes, and hypertension) this potential difference must be investigated further.[33]
Mental disorders play a role in individual differences in the quality and quantity of SWS: subjects suffering from depression show a lower amplitude of slow-wave activity compared to healthy participants. Sex differences also persist in the former group: depressed men present significantly lower SWA amplitude. This sex divergence is twice as large as the one observed in healthy subjects. However, no age-related difference concerning SWS can be observed in the depressed group.[34]
Brain regions
Some of the brain regions implicated in the induction of slow-wave sleep include:
- the parafacial zone (GABAergic neurons),[35][36][37] located within the medulla oblongata
- the nucleus accumbens core (GABAergic medium spiny neurons; specifically, the subset of these neurons that expresses both D2-type dopamine receptors and adenosine A2A receptors),[38][39][40] located within the striatum
- the ventrolateral preoptic area (GABAergic neurons),[36][37][41] located within the hypothalamus
- the lateral hypothalamus (melanin-concentrating hormone-releasing neurons),[36][37][42][43] located within the hypothalamus
Drugs
The chemical gamma-hydroxybutyric acid (GHB) has been studied to increase SWS.[44][45] In the United States, the Food and Drug Administration (FDA) permits the use of GHB under the trade name Xyrem to reduce cataplexy attacks and excessive daytime sleepiness in patients with narcolepsy.
See also
References
Notes
- Lesku, J. A.; Meyer, L. C. R.; Fuller, A.; Maloney, S. K.; Dell'Omo, G.; Vyssotski, A. L.; Rattenborg, N. C. (2011). Balaban, Evan (ed.). "Ostriches Sleep like Platypuses". PLOS ONE. 6 (8): e23203. Bibcode:2011PLoSO...623203L. doi:10.1371/journal.pone.0023203. PMC 3160860. PMID 21887239.
- Rechtschaffen, A; Kales, A (1968). A Manual of Standardized Terminology, Techniques and Scoring System For Sleep Stages of Human Subjects. US Dept of Health, Education, and Welfare; National Institutes of Health.
- Carlson, Neil R. (2012). Physiology of Behavior. Pearson. p. 291. ISBN 0205239390.
- Carlson, Neil R. (2012). Physiology of Behavior. Pearson. p. 291,293. ISBN 0205239390.
- Carey, Benedict (2013-01-27). "Aging in Brain Found to Hurt Sleep Needed for Memory". The New York Times.
- Walker, Matthew P. (1 January 2008). "Sleep-Dependent Memory Processing" (PDF). Harvard Review of Psychiatry. 16 (5): 287–298. doi:10.1080/10673220802432517. ISSN 1067-3229. PMID 18803104.
- http://walkerlab.berkeley.edu/reprints/Walker_JCSM_2009.pdf
- Walker, Matthew P. (15 April 2009). "The Role of Slow Wave Sleep in Memory Processing". Journal of Clinical Sleep Medicine. 5 (2 Suppl): S20–S26. doi:10.5664/jcsm.5.2S.S20. ISSN 1550-9389. PMC 2824214. PMID 19998871.
- Steriade, M. (1 January 2006). "Grouping of brain rhythms in corticothalamic systems" (PDF). Neuroscience. 137 (4): 1087–f1106. doi:10.1016/j.neuroscience.2005.10.029. PMID 16343791. S2CID 15470045.
- Gais, Steffen; Mölle, Matthias; Helms, Kay; Born, Jan (1 August 2002). "Learning-Dependent Increases in Sleep Spindle Density". Journal of Neuroscience. 22 (15): 6830–6834. doi:10.1523/JNEUROSCI.22-15-06830.2002. ISSN 0270-6474. PMID 12151563.
- http://www.architalbiol.org/aib/article/viewFile/411/370
- Rasch, Björn (9 Mar 2007). "Odor Cues During Slow-Wave Sleep Prompt Declarative Memory Consolidation". Science. 315 (5817): 1426–1429. Bibcode:2007Sci...315.1426R. doi:10.1126/science.1138581. PMID 17347444. S2CID 19788434.
- Peigneux, Philippe (28 October 2004). "Are Spatial Memories Strengthened in the Human Hippocampus during Slow Wave Sleep?". Neuron. 44 (3): 535–545. doi:10.1016/j.neuron.2004.10.007. PMID 15504332. S2CID 1424898.
- Scott, A. Cairney (1 April 2014). "Targeted Memory Reactivation During Slow Wave Sleep Facilitates Emotional Memory Consolidation". Sleep. 37 (4): 701–707. doi:10.5665/sleep.3572. PMC 3954173. PMID 24688163.
- Gais, Steffen (6 February 2004). "Low acetylcholine during slow-wave sleep is critical for declarative memory consolidation". PNAS. 101 (7): 2140–2144. Bibcode:2004PNAS..101.2140G. doi:10.1073/pnas.0305404101. PMC 357065. PMID 14766981.
- Carlson, Neil R. (2012). Physiology of Behavior. Pearson. pp. 297–298. ISBN 978-0205239399.
- Slow-Wave Sleep: Beyond Insomnia. Wolters Kluwer Pharma Solutions. ISBN 978-0-9561387-1-2.
- Schulz, Hartmut (2008). "Rethinking sleep analysis. Comment on the AASM Manual for the Scoring of Sleep and Associated Events". J Clin Sleep Med. 4 (2): 99–103. doi:10.5664/jcsm.27124. PMC 2335403. PMID 18468306.
Although the sequence of non-REM (NREM) sleep stages one to four (R&K classification) or N1 to N3 (AASM classification) fulfills the criteria...
- "Glossary. A resource from the Division of Sleep Medicine at Harvard Medical School, in partnership with WG Education Foundation". Harvard University. 2008. Retrieved 2009-03-11.
The 1968 categorization of the combined Sleep Stages 3 – 4 was reclassified in 2007 as Stage N3.
- Iber, C; Ancoli-Israel, S; Chesson, A; Quan, SF. for the American Academy of Sleep Medicine. The AASM Manual for the Scoring of Sleep and Associated Events: Rules, Terminology and Technical Specifications. Westchester: American Academy of Sleep Medicine; 2007.
- Jones, BE (1 May 2003). "Arousal systems". Frontiers in Bioscience. 8 (6): s438–51. doi:10.2741/1074. ISSN 1093-9946. PMID 12700104.
- Williams SR, Tóth TI, Turner JP, Hughes SW, Crunelli V (1997). "The 'window' component of the low threshold Ca2+ current produces input signal amplification and bistability in cat and rat thalamocortical neurones". The Journal of Physiology. 505 (Pt 3): 689–705. doi:10.1111/j.1469-7793.1997.689ba.x. PMC 1160046. PMID 9457646.
- Kattler, Herbert; Dijk, Derk-Jan; Borbely, Alexander A. (September 1994). "Effect of unilateral somatosensory stimulation prior to sleep on the sleep EEG in humans". Journal of Sleep Research. 3 (3): 159–164. doi:10.1111/j.1365-2869.1994.tb00123.x. PMID 10607121.
- Sekimoto, Masanori (1 May 2000). "Asymmetric interhemispheric delta waves during all-night sleep in humans". Clinical Neurophysiology. 111 (5): 924–928. doi:10.1016/S1388-2457(00)00258-3. PMID 10802465. S2CID 44808363.
- Tamaki, Masako; Bang, Ji Won; Watanabe, Takeo; Sasaki, Yuka (21 April 2016). "Night Watch in One Brain Hemisphere during Sleep Associated with the First-Night Effect in Humans". Current Biology. 26 (9): 1190–1194. doi:10.1016/j.cub.2016.02.063. PMC 4864126. PMID 27112296.
- Carlson, Neil R. (2012). Physiology of Behavior. Pearson. p. 305-307. ISBN 0205239390.
- "The importance of sleep & why we need it". 2015-10-23.
- Tononi, Giulio; Cirelli, Chiara (February 2006). "Sleep function and synaptic homeostasis". Sleep Medicine Reviews. 10 (1): 49–62. doi:10.1016/j.smrv.2005.05.002. PMID 16376591.
- Gulati, Tanuj; Guo, Ling; Ramanathan, Dhakshin S.; Bodepudi, Anitha; Ganguly, Karunesh (2017). "Neural reactivations during sleep determine network credit assignment". Nature Neuroscience. 20 (9): 1277–1284. doi:10.1038/nn.4601. PMC 5808917. PMID 28692062.
- Carlson, Neil R. (2012). Physiology of Behavior. Pearson. p. 298. ISBN 0205239390.
- Carlson, Neil R. (2012). Physiology of Behavior. Pearson. p. 299-300. ISBN 0205239390.
- Mander, Bryce A; Marks, Shawn M; Vogel, Jacob W; Rao, Vikram; Lu, Brandon; Saletin, Jared M; Ancoli-Israel, Sonia; Jagust, William J; Walker, Matthew P (1 June 2015). "β-amyloid disrupts human NREM slow waves and related hippocampus-dependent memory consolidation". Nature Neuroscience. 18 (7): 1051–1057. doi:10.1038/nn.4035. PMC 4482795. PMID 26030850.
- Mokhlesi, Babak; Pannain, Silvana; Ghods, Farbod; Knutson, Kristen L. (2012). "Predictors of slow-wave sleep in a clinic-based sample". Journal of Sleep Research. 21 (2): 170–175. doi:10.1111/j.1365-2869.2011.00959.x. PMC 3321544. PMID 21955220.
- Armitage, Roseanne (11 July 2000). "Slow-wave activity in NREM sleep: sex and age effects in depressed outpatients and healthy controls". Psychiatry Research. 95 (3): 201–213. doi:10.1016/S0165-1781(00)00178-5. PMID 10974359. S2CID 1903649.
- Anaclet C, Ferrari L, Arrigoni E, Bass CE, Saper CB, Lu J, Fuller PM (September 2014). "The GABAergic parafacial zone is a medullary slow wave sleep-promoting center" (PDF). Nat. Neurosci. 17 (9): 1217–1224. doi:10.1038/nn.3789. PMC 4214681. PMID 25129078.
In the present study we show, for the first time, that activation of a delimited node of GABAergic neurons located in the medullary PZ can potently initiate SWS and cortical SWA in behaving animals. ... For now however it remains unclear if the PZ is interconnected with other sleep– and wake–promoting nodes beyond the wake–promoting PB. ... The intensity of cortical slow–wave–activity (SWA: 0.5–4Hz) during SWS is also widely accepted as a reliable indicator of sleep need ... In conclusion, in the present study we demonstrated that all polygraphic and neurobehavioral manifestation of SWS, including SWA, can be initiated in behaving animals by the selective activation of a delimited node of GABAergic medullary neurons.
- Schwartz MD, Kilduff TS (December 2015). "The Neurobiology of Sleep and Wakefulness". The Psychiatric Clinics of North America. 38 (4): 615–644. doi:10.1016/j.psc.2015.07.002. PMC 4660253. PMID 26600100.
More recently, the medullary parafacial zone (PZ) adjacent to the facial nerve was identified as a sleep-promoting center on the basis of anatomical, electrophysiological and chemo- and optogenetic studies.23, 24 GABAergic PZ neurons inhibit glutamatergic parabrachial (PB) neurons that project to the BF,25 thereby promoting NREM sleep at the expense of wakefulness and REM sleep. ... Sleep is regulated by GABAergic populations in both the preoptic area and the brainstem; increasing evidence suggests a role for the melanin-concentrating hormone cells of the lateral hypothalamus and the parafacial zone of the brainstem
- Brown RE, McKenna JT (June 2015). "Turning a Negative into a Positive: Ascending GABAergic Control of Cortical Activation and Arousal". Front. Neurol. 6: 135. doi:10.3389/fneur.2015.00135. PMC 4463930. PMID 26124745.
The sleep-promoting action of GABAergic neurons located in the preoptic hypothalamus (6–8) is now well-known and accepted (9). More recently, other groups of sleep-promoting GABAergic neurons in the lateral hypothalamus (melanin-concentrating hormone neurons) and brainstem [parafacial zone; (10)] have been identified.
- Valencia Garcia S, Fort P (December 2017). "Nucleus Accumbens, a new sleep-regulating area through the integration of motivational stimuli". Acta Pharmacologica Sinica. 39 (2): 165–166. doi:10.1038/aps.2017.168. PMC 5800466. PMID 29283174.
The nucleus accumbens comprises a contingent of neurons specifically expressing the post-synaptic A2A-receptor (A2AR) subtype making them excitable by adenosine, its natural agonist endowed with powerful sleep-promoting properties[4]. ... In both cases, large activation of A2AR-expressing neurons in NAc promotes slow wave sleep (SWS) by increasing the number and duration of episodes. ... After optogenetic activation of the core, a similar promotion of SWS was observed, whereas no significant effects were induced when activating A2AR-expressing neurons within the shell.
- Oishi Y, Xu Q, Wang L, Zhang BJ, Takahashi K, Takata Y, Luo YJ, Cherasse Y, Schiffmann SN, de Kerchove d'Exaerde A, Urade Y, Qu WM, Huang ZL, Lazarus M (September 2017). "Slow-wave sleep is controlled by a subset of nucleus accumbens core neurons in mice". Nature Communications. 8 (1): 734. Bibcode:2017NatCo...8..734O. doi:10.1038/s41467-017-00781-4. PMC 5622037. PMID 28963505.
Here, we show that chemogenetic or optogenetic activation of excitatory adenosine A2A receptor-expressing indirect pathway neurons in the core region of the NAc strongly induces slow-wave sleep. Chemogenetic inhibition of the NAc indirect pathway neurons prevents the sleep induction, but does not affect the homoeostatic sleep rebound.
- Yuan XS, Wang L, Dong H, Qu WM, Yang SR, Cherasse Y, Lazarus M, Schiffmann SN, d'Exaerde AK, Li RX, Huang ZL (October 2017). "Striatal adenosine A2A receptor neurons control active-period sleep via parvalbumin neurons in external globus pallidus". eLife. 6: e29055. doi:10.7554/eLife.29055. PMC 5655138. PMID 29022877.
- Varin C, Rancillac A, Geoffroy H, Arthaud S, Fort P, Gallopin T (2015). "Glucose Induces Slow-Wave Sleep by Exciting the Sleep-Promoting Neurons in the Ventrolateral Preoptic Nucleus: A New Link between Sleep and Metabolism". The Journal of Neuroscience. 35 (27): 9900–11. doi:10.1523/JNEUROSCI.0609-15.2015. PMC 6605416. PMID 26156991.
- Monti JM, Torterolo P, Lagos P (2013). "Melanin-concentrating hormone control of sleep-wake behavior". Sleep Medicine Reviews. 17 (4): 293–8. doi:10.1016/j.smrv.2012.10.002. PMID 23477948.
MCHergic neurons are silent during wakefulness (W), increase their firing during slow wave sleep (SWS) and still more during REM sleep (REMS). Studies in knockout mice for MCH (MCH(-/-)) have shown a reduction in SWS and an increase of W during the light and the dark phase of the light-dark cycle.
- Torterolo P, Lagos P, Monti JM (2011). "Melanin-concentrating hormone: a new sleep factor?". Frontiers in Neurology. 2: 14. doi:10.3389/fneur.2011.00014. PMC 3080035. PMID 21516258.
Neurons containing the neuropeptide melanin-concentrating hormone (MCH) are mainly located in the lateral hypothalamus and the incerto-hypothalamic area, and have widespread projections throughout the brain. ... Intraventricular microinjection of MCH increases both slow wave sleep (SWS) and REM sleep; however, the increment in REM sleep is more pronounced. ... Although both SWS and REM sleep are facilitated by MCH, REM sleep seems to be more sensitive to MCH modulation.
- Roehrs, Timothy; Roth, Thomas (December 2010). "Drug-Related Sleep Stage Changes: Functional Significance and Clinical Relevance". Sleep Medicine Clinics. 5 (4): 559–570. doi:10.1016/j.jsmc.2010.08.002. PMC 3041980. PMID 21344068.
- "Xyrem - European Drugs Reference Encyclopedia".
Further reading
- M. Massimini, G. Tononi, et al., "Breakdown of Cortical Effective Connectivity During Sleep," Science, vol. 309, 2005, pp. 2228–32.
- P. Cicogna, V. Natale, M. Occhionero, and M. Bosinelli, "Slow Wave and REM Sleep Mentation," Sleep Research Online, vol. 3, no. 2, 2000, pp. 67–72.
- D. Foulkes et al., "Ego Functions and Dreaming During Sleep Onset," in Charles Tart, ed., Altered States of Consciousness, p. 75.
- Rock, Andrea (2004). The Mind at Night.
- Warren, Jeff (2007). "The Slow Wave". The Head Trip: Adventures on the Wheel of Consciousness. ISBN 978-0-679-31408-0.