Enhanced weathering
Enhanced weathering or accelerated weathering refers to geoengineering approaches intended to remove carbon dioxide from the atmosphere by using of specific natural or artificially created minerals which absorb carbon dioxide and transform it in other substances through chemical reactions occurring in the presence of water (for example in the form of rain, groundwater or seawater).
Enhanced weathering research considers how natural processes of rocks' and minerals' weathering (in particular chemical weathering) may be enhanced to sequester CO2 from the atmosphere to be stored in form of another substance in solid carbonate minerals or ocean alkalinity. Since the carbon dioxide is usually first removed from ocean water, these approaches would attack the problem by first reducing ocean acidification.
This technique requires the extraction or production of large quantities of materials, crushing them and spreading them over large areas (for example fields or beaches); for this reason, in comparison with other methods of removing carbon dioxide from the atmosphere currently available (reforestation and BECCS - Bio-Energy with Carbon Capture and Storage), it is particularly expensive. It also has the side effect of altering the natural salinity of the seas.
History
The approach is largely theoretical proposed for both terrestrial and ocean based sequestration. Ocean methods are being tested by non-profit Project Vesta to see if it is a environmentally and economically viable carbon sequestration strategy.[1][2]
In July 2020 a group of scientists assessed that the geoengineering technique of enhanced rock weathering – spreading finely crushed basalt on fields – has potential use for carbon dioxide removal by nations, identifying costs, opportunities and engineering challenges.[3][4]
Natural mineral weathering and ocean acidification mechanism
_28.jpg.webp)
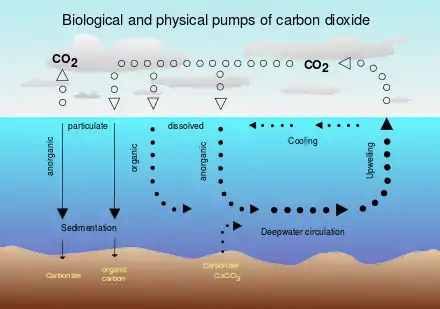
Weathering is the natural process in which rocks and minerals are broken down and dissolved on the land surface because of the action of water, ice, acids, salts, plants, animals, and changes in temperature.[5] It is distinguished between mechanical weathering (or physical weathering or disaggregation) and chemical weathering (which transforms the chemical nature of the rocks).[5] Biological weathering is a form of weathering (mechanical and/or chemical) due to the action of plant, fungi or others living organisms.[5]
Chemical weathering can happen with different mechanism, depending mainly on the nature of the mineral involved: solution, hydration, hydrolysis and oxidation weathering.[6] Carbonation weathering is a particular type of solution weathering.[6]
Carbonate and silicate minerals are examples of minerals affected by carbonation weathering. When silicate or carbonate minerals are exposed to rainwater or groundwater, they slowly dissolve because of chemical weathering associated with the reaction of carbonation, that means the combination of the water (H2O) and carbon dioxide (CO2) present in the atmosphere to form carbonic acid (H2CO3):[5][7]
- H2O + CO2 → H2CO3
Carbonic acid consequently attacks the mineral to form carbonate ions in solution with the unreacted water. Because of these two chemical reactions (carbonation and dissolution), mineral, water and carbon dioxide combine together altering the chemical composition of minerals and diminishing the presence of CO2 in the atmosphere.
In particular, forsterite (a silicate mineral) is dissolved through the reaction:
- Mg2SiO4(s) + 4H2CO3(aq) → 2Mg2+(aq) + 4HCO3−(aq) + H4SiO4(aq)
Where "(s)" indicates a substance in a solid state and "(aq)" indicates a substance in an aqueous solution.
Calcite (a carbonate mineral) is instead dissolved through the reaction:
- CaCO3(s) + H2CO3(aq) → Ca2+(aq) + 2HCO3−(aq)
Water with dissolved bicarbonate ions (HCO3−) eventually ends up in the ocean,[7] where bicarbonate ions form carbonate minerals by calcifying organisms through the reaction:
- Ca2+ + 2HCO3− → CaCO3 + CO2 + H2O
The carbonate minerals then eventually sink from the ocean surface to the ocean floor.[7] Most of the carbonate is redissolved in the deep ocean as it sinks.
Over geological time periods these processes are thought to stabilise the Earth's climate.[8] In fact the quantity of carbon dioxide in the atmosphere as a gas (CO2) respect to the quantity of carbon dioxide converted into carbonate is regulated by a chemical equilibrium: in case of a change of this equilibrium state, it takes theoretically (that means if no other alteration is happening during time) thousand of years to establish a new equilibrium state.[7]
For silicate weathering the theoretical net effect of dissolution and precipitation is 1 mol of CO2 sequestered for every mol of Ca2+ or Mg2+ weathered out of the mineral. Given that some of the dissolved cations react with existing alkalinity in the solution to form CO32− ions, the ratio is not exactly 1:1 in natural systems but is a function of temperature and CO2 partial pressure. The net CO2 sequestration of carbonate weathering reaction and carbonate precipitation reaction is zero.

Weathering and biological carbonate precipitation are thought to be only loosely coupled on short time periods (<1000 years). Therefore, an increase in both carbonate and silicate weathering with respect to carbonate precipitation will result in a buildup of alkalinity in the ocean.
Terrestrial enhanced weathering
Enhanced weathering was initially used to refer specifically to the spreading of crushed silicate minerals on the land surface.[9][10] Biological activity in soils has been shown to promote the dissolution of silicate minerals (see discussion in,[11] but there is still uncertainty surrounding how quickly this may happen. As weathering rate is a function of saturation of the dissolving mineral in solution (decreasing to zero in fully saturated solutions), some have suggested that the quantity of rainfall may limit terrestrial enhanced weathering,[12] although others[13] suggest that secondary mineral formation or biological uptake may suppress saturation and promote weathering.
The amount of energy that is required for comminution depends on rate at which the minerals dissolve (less comminution is required for rapid mineral dissolution). Recent work[14] has suggested a large range in potential cost of enhanced weathering largely down to the uncertainty surrounding mineral dissolution rates.
Oceanic enhanced weathering
To overcome the limitations of solution saturation and to use natural comminution of sand particles from wave energy, silicate minerals may be applied to coastal environments,[15] although the higher pH of seawater may substantially decrease the rate of dissolution,[16] and it is unclear how much comminution is possible from wave action.
Alternatively, the direct application of carbonate minerals to the up-welling regions of the ocean has been investigated.[17] Carbonate minerals are supersaturated in the surface ocean but are undersaturated in the deep ocean. In areas of up welling this undersaturated water is brought to the surface. While this technology will likely be cheap, the maximum annual CO2 sequestration potential is limited.
Transforming the carbonate minerals into oxides and spreading this material in the open ocean ('Ocean Liming') has been proposed as an alternative technology.[18] Here the carbonate mineral (CaCO3) is transformed into lime (CaO) through calcination. The energy requirements for this technology are substantial.
Mineral carbonation
The enhanced dissolution and carbonation of silicates ('mineral carbonation') was first proposed by Seifritz,[19] and developed initially by Lackner et al.[20] and further by the Albany Research Center.[21] This early research investigated the carbonation of extracted and crushed silicates at elevated temperatures (~180 °C) and partial pressures of CO2 (~15 MPa) inside controlled reactors ('Ex-situ mineral carbonation'). Some research explores the potential of 'In-situ mineral carbonation' in which the CO2 is injected into silicate rock formations to promote carbonate formation underground (see: CarbFix)
Mineral carbonation research has largely focused on the sequestration of CO2 from flue gas. It could be used for geoengineering if the source of CO2 was derived from the atmosphere, e.g. through direct air capture or biomass-CCS.
See also
References
- Peters, Adele (2020-05-29). "Ever been to a green sand beach? The newest geohack to fight climate change". Fast Company. Retrieved 2020-11-06.
- Delbert, Caroline (2020-06-11). "How This Strange Green Sand Could Reverse Climate Change". Popular Mechanics. Retrieved 2020-11-06.
- "Applying rock dust to croplands could absorb up to 2 billion tonnes of CO2 from the atmosphere". phys.org. Retrieved 16 August 2020.
- Beerling, David J.; Kantzas, Euripides P.; Lomas, Mark R.; Wade, Peter; Eufrasio, Rafael M.; Renforth, Phil; Sarkar, Binoy; Andrews, M. Grace; James, Rachael H.; Pearce, Christopher R.; Mercure, Jean-Francois; Pollitt, Hector; Holden, Philip B.; Edwards, Neil R.; Khanna, Madhu; Koh, Lenny; Quegan, Shaun; Pidgeon, Nick F.; Janssens, Ivan A.; Hansen, James; Banwart, Steven A. (July 2020). "Potential for large-scale CO 2 removal via enhanced rock weathering with croplands". Nature. 583 (7815): 242–248. doi:10.1038/s41586-020-2448-9. ISSN 1476-4687. Archived from the original on 16 Jul 2020. Retrieved 16 August 2020.
- National Geographic - Weathering
- Brandon Vogt, "Rock Weathering"
- Encyclopædia Britannica - Biological carbon cycle
- Berner, Robert A. Berner; Kothavala, Zavareth (2001). "GEOCARB III: A revised model of atmospheric CO2 over Phanerozoic time". American Journal of Science. 301 (2): 182–204. Bibcode:2001AmJS..301..182B. CiteSeerX 10.1.1.393.582. doi:10.2475/ajs.301.2.182.
- Schuiling, R. D.; Krijgsman, P. (2006). "Enhanced Weathering: An Effective and Cheap Tool to Sequester CO2". Climatic Change. 74 (1–3): 349–54. doi:10.1007/s10584-005-3485-y.
- Manning, D. A. C. (2008). "Biological enhancement of soil carbonate precipitation: Passive removal of atmospheric CO2". Mineralogical Magazine. 72 (2): 639–49. Bibcode:2008MinM...72..639M. doi:10.1180/minmag.2008.072.2.639.
- Manning, David A. C.; Renforth, Phil (2013). "Passive Sequestration of Atmospheric CO2 through Coupled Plant-Mineral Reactions in Urban soils". Environmental Science & Technology. 47 (1): 135–41. Bibcode:2013EnST...47..135M. doi:10.1021/es301250j. PMID 22616942.
- Köhler, Peter; Hartmann, Jens; Wolf-Gladrow, Dieter A.; Schellnhuber, Hans-Joachim (2010). "Geoengineering potential of artificially enhanced silicate weathering of olivine". Proceedings of the National Academy of Sciences. 107 (47): 20228–33. Bibcode:2010EGUGA..12.6986K. doi:10.1073/pnas.1000545107. JSTOR 25756680. PMC 2996662. PMID 21059941.
- Schuiling, Roelof D.; Wilson, Siobhan A.; Power, lan M. (2011). "Enhanced silicate weathering is not limited by silicic acid saturation". Proceedings of the National Academy of Sciences. 108 (12): E41. Bibcode:2011PNAS..108E..41S. doi:10.1073/pnas.1019024108. PMC 3064366. PMID 21368192.
- Renforth, P. (2012). "The potential of enhanced weathering in the UK" (PDF). International Journal of Greenhouse Gas Control. 10: 229–43. doi:10.1016/j.ijggc.2012.06.011.
- Schuiling, R.D.; de Boer, P.L. (2010). "Coastal spreading of olivine to control atmospheric CO2 concentrations: A critical analysis of viability. Comment: Nature and laboratory models are different". International Journal of Greenhouse Gas Control. 4 (5): 855–6. doi:10.1016/j.ijggc.2010.04.012.
- Hangx, Suzanne J.T.; Spiers, Christopher J. (2009). "Coastal spreading of olivine to control atmospheric CO2 concentrations: A critical analysis of viability". International Journal of Greenhouse Gas Control. 3 (6): 757–67. doi:10.1016/j.ijggc.2009.07.001.
- Harvey, L. D. D. (2008). "Mitigating the atmospheric CO2 increase and ocean acidification by adding limestone powder to upwelling regions". Journal of Geophysical Research. 113 (C4): C04028. Bibcode:2008JGRC..113.4028H. doi:10.1029/2007JC004373.
- Kheshgi, Haroon S. (1995). "Sequestering atmospheric carbon dioxide by increasing ocean alkalinity". Energy. 20 (9): 915–22. doi:10.1016/0360-5442(95)00035-F.
- Seifritz, W. (1990). "CO2 disposal by means of silicates". Nature. 345 (6275): 486. Bibcode:1990Natur.345..486S. doi:10.1038/345486b0.
- Lackner, Klaus S.; Wendt, Christopher H.; Butt, Darryl P.; Joyce, Edward L.; Sharp, David H. (1995). "Carbon dioxide disposal in carbonate minerals". Energy. 20 (11): 1153. doi:10.1016/0360-5442(95)00071-N.
- O’Connor, W. K.; Dahlin, D. C.; Rush, G. E.; Gedermann, S. J.; Penner, L. R.; Nilsen, D. N. (March 15, 2005). Aqueous mineral carbonation, Final Report (PDF). National Energy Technology Laboratory.