Carbon dioxide removal
Carbon dioxide removal (CDR), also known as greenhouse gas removal, is a process in which carbon dioxide gas (CO
2) is removed from the atmosphere and sequestered for long periods of time.[1][2][3] These methods are also known as negative emissions technologies, as they offset greenhouse gas emissions from practices such as the burning of fossil fuels.[4]
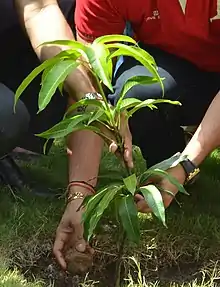
CDR methods include afforestation, agricultural practices that sequester carbon in soils, bio-energy with carbon capture and storage, ocean fertilization, enhanced weathering, and direct air capture when combined with storage.[2][5][6]To assess whether net negative emissions are achieved by a particular process, comprehensive life cycle analysis of the process must be performed.
Alternatively, some sources use the term "carbon dioxide removal" to refer to any technology that removes carbon dioxide, such as direct air capture, but can be implemented in a way that causes emissions to increase rather than decrease over the lifecycle of the process.
The IPCC's analysis of climate change mitigation pathways that are consistent with limiting global warming to 1.5°C found that all assessed pathways include the use of CDR to offset emissions.[7] A 2019 consensus report by NASEM concluded that using existing CDR methods at scales that can be safely and economically deployed, there is potential to remove and sequester up to 10 gigatons of carbon dioxide per year.[4] This would offset greenhouse gas emissions at about a fifth of the rate at which they are being produced.
Definitions
Part of a series on the |
Carbon cycle |
---|
![]() |
The Intergovernmental Panel on Climate Change defines CDR as:
Anthropogenic activities removing CO
2 from the atmosphere and durably storing it in geological, terrestrial, or ocean reservoirs, or in products. It includes existing and potential anthropogenic enhancement of biological or geochemical sinks and direct air capture and storage, but excludes natural CO
2 uptake not directly caused by human activities.[1]
The U.S.-based National Academies of Sciences, Engineering, and Medicine (NASEM) uses the term "negative emissions technology" with a similar definition.[4]
The concept of deliberately reducing the amount of CO
2 in the atmosphere is often mistakenly classified with solar radiation management as a form of climate engineering and assumed to be intrinsically risky.[4] In fact, CDR addresses the root cause of climate change and is part of strategies to reduce net emissions.[2]
Concepts using similar terminology
CDR can be confused with carbon capture and storage (CCS), a process in which carbon dioxide is collected from point-sources such as gas-fired power plants, whose smokestacks emit CO2 in a concentrated stream. The CO2 is then compressed and sequestered or utilized.[1] When used to sequester the carbon from a gas-fired power plant, CCS reduces emissions from continued use of the point source, but does not reduce the amount of carbon dioxide already in the atmosphere.
Potential for climate change mitigation
Using CDR in parallel with other efforts to reduce greenhouse gas emissions, such as deploying renewable energy, is likely to be less expensive and disruptive than using other efforts alone.[4] A 2019 consensus study report by NASEM assessed the potential of all forms of CDR other than ocean fertilization that could be deployed safely and economically using current technologies, and estimated that they could remove up to 10 gigatons of CO
2 per year if fully deployed worldwide.[4] This is one-fifth of the 50 gigatons of CO
2 emitted per year by human activities.[4] In the IPCC's 2018 analysis of ways to limit climate change, all analyzed mitigation pathways that would prevent more than 1.5°C of warming included CDR measures.[8]
Some mitigation pathways propose achieving higher rates of CDR through massive deployment of one technology, however these pathways assume that hundreds of millions of hectares of cropland are converted to growing biofuel crops.[4] Further research in the areas of direct air capture, geologic sequestration of carbon dioxide, and carbon mineralization could potentially yield technological advancements that make higher rates of CDR economically feasible.[4]
The IPCC's 2018 report said that reliance on large-scale deployment of CDR would be a "major risk" to achieving the goal of less than 1.5°C of warming, given the uncertainties in how quickly CDR can be deployed at scale.[8] Strategies for mitigating climate change that rely less on CDR and more on sustainable use of energy carry less of this risk.[8][9] The possibility of large-scale future CDR deployment has been described as a moral hazard, as it could lead to a reduction in near-term efforts to mitigate climate change.[10][4] The 2019 NASEM report concludes:
Any argument to delay mitigation efforts because NETs will provide a backstop drastically misrepresents their current capacities and the likely pace of research progress.[4]
Carbon sequestration
Forests, kelp beds, and other forms of plant life absorb carbon dioxide from the air as they grow, and bind it into biomass. As the use of plants as carbon sinks can be undone by events such as wildfires, the long-term reliability of these approaches has been questioned.
Carbon dioxide that has been removed from the atmosphere can also be stored in the Earth's crust by injecting it into the subsurface, or in the form of insoluble carbonate salts (mineral sequestration). This is because they are removing carbon from the atmosphere and sequestering it indefinitely and presumably for a considerable duration (thousands to millions of years). Carbon capture technology has yet to reach more than 33% efficiency.
Methods
Afforestation, reforestation, and forestry management
According to the International Union for Conservation of Nature: "Halting the loss and degradation of natural systems and promoting their restoration have the potential to contribute over one-third of the total climate change mitigation scientists say is required by 2030."[11]
Biosequestration
Biosequestration is the capture and storage of the atmospheric greenhouse gas carbon dioxide by continual or enhanced biological processes. This form of carbon sequestration occurs through increased rates of photosynthesis via land-use practices such as reforestation, sustainable forest management, and genetic engineering. The SALK Harnessing Plants Initiative lead by Joanne Chory is an example of an enhanced photosynthesis initiative[12][13]
Carbon sequestration through biological processes affects the global carbon cycle. Examples include major climatic fluctuations, such as the Azolla event, which created the current Arctic climate. Such processes created fossil fuels, as well as clathrate and limestone. By manipulating such processes, geoengineers seek to enhance sequestration
Agricultural practices
Carbon farming is a name for a variety of agricultural methods aimed at sequestering atmospheric carbon into the soil and in crop roots, wood and leaves. Increasing a soil's organic matter content can aid plant growth, increase total carbon content, improve soil water retention capacity[14] and reduce fertilizer use[15].[16] As of 2016, variants of carbon farming reached hundreds of millions of hectares globally, of the nearly 5 billion hectares (1.2×1010 acres) of world farmland.[17] Soils can contain up to five per cent carbon by weight, including decomposing plant and animal matter and biochar.[18]
Potential sequestration alternatives to carbon farming include scrubbing CO2 from the air with machines (direct air capture); fertilizing the oceans to prompt algal blooms that after death carry carbon to the sea bottom[19];storing the carbon dioxide emitted by electricity generation; and crushing and spreading types of rock such as basalt that absorb atmospheric carbon.[16] Land management techniques that can be combined with farming include planting/restoring forests, burying biochar produced by anaerobically converted biomass and restoring wetlands. (Coal beds are the remains of marshes and peatlands.)[20]Wetland restoration
Blue carbon refers to carbon dioxide removed from the atmosphere by the world's coastal ocean ecosystems, mostly mangroves, salt marshes, seagrasses and macroalgae, through plant growth and the accumulation and burial of organic matter in the soil.[21][23][24]
Historically the ocean, atmosphere, soil, and terrestrial forest ecosystems have been the largest natural carbon (C) sinks. New research on the role of vegetated coastal ecosystems has highlighted their potential as highly efficient C sinks,[25] and led to the scientific recognition of the term "Blue Carbon".[26] "Blue Carbon" designates carbon that is fixed via coastal ocean ecosystems, rather than traditional land ecosystems, like forests. Although the ocean's vegetated habitats cover less than 0.5% of the seabed, they are responsible for more than 50%, and potentially up to 70%, of all carbon storage in ocean sediments.[26] Mangroves, salt marshes and seagrasses make up the majority of the ocean's vegetated habitats but only equal 0.05% of the plant biomass on land. Despite their small footprint, they can store a comparable amount of carbon per year and are highly efficient carbon sinks. Seagrasses, mangroves and salt marshes can capture carbon dioxide (CO
2) from the atmosphere by sequestering the C in their underlying sediments, in underground and below-ground biomass, and in dead biomass.[27][28]
Bio-energy with carbon capture & storage
Bio-energy with carbon capture and storage, or BECCS, uses biomass to extract carbon dioxide from the atmosphere, and carbon capture and storage technologies to concentrate and permanently store it in deep geological formations.
BECCS is currently (as of October 2012) the only CDR technology deployed at full industrial scale, with 550 000 tonnes CO2/year in total capacity operating, divided between three different facilities (as of January 2012).[29][30][31][32][33]
Imperial College London, the UK Met Office Hadley Centre for Climate Prediction and Research, the Tyndall Centre for Climate Change Research, the Walker Institute for Climate System Research, and the Grantham Institute for Climate Change issued a joint report on carbon dioxide removal technologies as part of the AVOID: Avoiding dangerous climate change research program, stating that "Overall, of the technologies studied in this report, BECCS has the greatest maturity and there are no major practical barriers to its introduction into today's energy system. The presence of a primary product will support early deployment."[34]
According to the OECD, "Achieving lower concentration targets (450 ppm) depends significantly on the use of BECCS".[35]
Biochar
Biochar is created by the pyrolysis of biomass, and is under investigation as a method of carbon sequestration. Biochar is a charcoal that is used for agricultural purposes which also aids in carbon sequestration, the capture or hold of carbon. It is created using a process called pyrolysis, which is basically the act of high temperature heating biomass in an environment with low oxygen levels. What remains is a material known as char, similar to charcoal but is made through a sustainable process, thus the use of biomass.[36] Biomass is organic matter produced by living organisms or recently living organisms, most commonly plants or plant based material.[37] The offset of greenhouse gas (GHG) emission, if biochar were to be implemented, would be a maximum of 12%. This equates to about 106 metric tons of CO2 equivalents. On a medium conservative level, it would be 23% less than that, at 82 metric tons.[38] A study done by the UK Biochar Research Center has stated that, on a conservative level, biochar can store 1 gigaton of carbon per year. With greater effort in marketing and acceptance of biochar, the benefit could be the storage of 5–9 gigatons per year of carbon in biochar soils.[39]
Enhanced weathering
Enhanced weathering is a chemical approach to remove carbon dioxide involving land- or ocean-based techniques. One example of a land-based enhanced weathering technique is in-situ carbonation of silicates. Ultramafic rock, for example, has the potential to store from hundreds to thousands of years' worth of CO2 emissions, according to estimates.[40][41] Ocean-based techniques involve alkalinity enhancement, such as grinding, dispersing, and dissolving olivine, limestone, silicates, or calcium hydroxide to address ocean acidification and CO2 sequestration. Enhanced weathering is considered one of the least expensive geoengineering options. One example of a research project on the feasibility of enhanced weathering is the CarbFix project in Iceland.[42][43][44]
Direct air capture
Direct air capture (DAC) is the use of chemical or physical processes to extract CO
2 directly from the ambient air.[45] If the extracted CO
2 is then sequestered in safe long-term storage, the overall process will achieve carbon dioxide removal.
A few engineering proposals have been made for DAC, but work in this area is still in its infancy.[46] A private company Global Research Technologies demonstrated a pre-prototype of air capture technology in 2007.[47] Carbon Engineering is a commercial DAC company founded in 2009 and backed, among others, by Bill Gates and Murray Edwards.[48][49] As of 2018, they run a pilot plant in British Columbia, Canada that has been in use since 2015[50] and is able to extract about a tonne of CO
2 a day.[51][49] An economic study of their pilot plant conducted from 2015 to 2018 estimated the cost at $94–232 per tonne of atmospheric CO
2 removed.[50][52] Several companies such as Zurich startup Climeworks and California based Prometheus Fuels are now working on this approach.
DAC relying on amine-based absorption demands significant water input. It was estimated, that to capture 3.3 Gigatonnes of CO
2 a year would require 300 km3 of water, or 4% of the water used for irrigation. On the other hand, using sodium hydroxide needs far less water, but the substance itself is highly caustic and dangerous.[53]
DAC also requires much greater energy input in comparison to traditional capture from point sources, like flue gas, due to the low concentration of CO
2.[54][55] The theoretical minimum energy required to extract CO
2 from ambient air is about 250 kWh per tonne of CO
2, while capture from natural gas and coal power plants requires respectively about 100 and 65 kWh per tonne of CO
2.[56]
Ocean fertilization
Economic issues
A crucial issue for CDR is the cost, which differs substantially among the different methods: some of these are not sufficiently developed to perform cost assessments. A 2018 study estimated the cost of direct air capture as between $94 and $232 per tonne.[60][61] However the value of BECCS and CDR generally in integrated assessment models in the long term is highly dependant on the discount rate.[62]
On 21 January 2021, Elon Musk announced he was donating $100m for a prize for best carbon capture technology.[63]
Removal of other greenhouse gases
Although some researchers have suggested methods for removing methane, others say that nitrous oxide would be a better subject for research due to its longer lifetime in the atmosphere.[64]
Bibliography
- IPCC, 2018: Global Warming of 1.5°C. An IPCC Special Report on the impacts of global warming of 1.5°C above pre-industrial levels and related global greenhouse gas emission pathways, in the context of strengthening the global response to the threat of climate change, sustainable development, and efforts to eradicate poverty [V. Masson-Delmotte, P. Zhai, H. O. Pörtner, D. Roberts, J. Skea, P.R. Shukla, A. Pirani, W. Moufouma-Okia, C. Péan, R. Pidcock, S. Connors, J. B. R. Matthews, Y. Chen, X. Zhou, M. I. Gomis, E. Lonnoy, T. Maycock, M. Tignor, T. Waterfield (eds.)].
- Report website, chapters I–V
- Summary for policymakers, 32 pp.
- Headline statements, 2 pp.
- Technical summary, 22 pp.
- FAQ, 24 pp.
- Glossary, 24 pp.
See also
References
- Intergovernmental Panel on Climate Change. "Glossary — Global Warming of 1.5 ºC". Retrieved February 23, 2020.
- "Geoengineering the climate: science, governance and uncertainty". The Royal Society. 2009. Retrieved September 10, 2011.
- Minx, Jan C; Lamb, William F; Callaghan, Max W; Fuss, Sabine; Hilaire, Jérôme; Creutzig, Felix; Amann, Thorben; Beringer, Tim; De Oliveira Garcia, Wagner; Hartmann, Jens; Khanna, Tarun; Lenzi, Dominic; Luderer, Gunnar; Nemet, Gregory F; Rogelj, Joeri; Smith, Pete; Vicente Vicente, Jose Luis; Wilcox, Jennifer; Del Mar Zamora Dominguez, Maria (2018). "Negative emissions: Part 1 – research landscape and synthesis" (PDF). Environmental Research Letters. 13 (6): 063001. Bibcode:2018ERL....13f3001M. doi:10.1088/1748-9326/aabf9b.
- National Academies of Sciences, Engineering (October 24, 2018). Negative Emissions Technologies and Reliable Sequestration: A Research Agenda. ISBN 978-0-309-48452-7.
- Vergragt, P.J.; Markusson, N.; Karlsson, H. (2011). "Carbon capture and storage, bio-energy with carbon capture and storage, and the escape from the fossil-fuel lock-in". Global Environmental Change. 21 (2): 282–92. doi:10.1016/j.gloenvcha.2011.01.020.
- Azar, C.; Lindgren, K.; Larson, E.; Möllersten, K. (2006). "Carbon Capture and Storage from Fossil Fuels and Biomass – Costs and Potential Role in Stabilizing the Atmosphere". Climatic Change. 74 (1–3): 47–79. Bibcode:2006ClCh...74...47A. doi:10.1007/s10584-005-3484-7.
- IPCC15, Ch 2.
- "SR15 Technical Summary" (PDF). Retrieved July 25, 2019.
- Anderson, K.; Peters, G. (October 14, 2016). "The trouble with negative emissions". Science. 354 (6309): 182–183. doi:10.1126/science.aah4567. hdl:11250/2491451. ISSN 0036-8075.
- IPCC15 & Ch. 2 p. 124.
- "Forests and climate change". IUCN. November 11, 2017. Retrieved October 7, 2020.
- Beerling, David (2008). The Emerald Planet: How Plants Changed Earth's History. Oxford University Press. pp. 194–5. ISBN 978-0-19-954814-9.
- National Academies Of Sciences, Engineering (2019). Negative Emissions Technologies and Reliable Sequestration: A Research Agenda. Washington, D.C.: National Academies of Sciences, Engineering, and Medicine. pp. 45–136. doi:10.17226/25259. ISBN 978-0-309-48452-7. PMID 31120708.
- "Carbon Farming | Carbon Cycle Institute". www.carboncycle.org. Retrieved April 27, 2018.
- "Carbon Farming: Hope for a Hot Planet – Modern Farmer". Modern Farmer. March 25, 2016. Retrieved April 25, 2018.
- Velasquez-Manoff, Moises (April 18, 2018). "Can Dirt Save the Earth?". The New York Times. ISSN 0362-4331. Retrieved April 28, 2018.
- "Excerpt | The Carbon Farming Solution". carbonfarmingsolution.com. Retrieved April 27, 2018.
- Burton, David. "How carbon farming can help solve climate change". The Conversation. Retrieved April 27, 2018.
- Ortega, Alejandra; Geraldi, N.R.; Alam, I.; Kamau, A.A.; Acinas, S.; Logares, R.; Gasol, J.; Massana, R.; Krause-Jensen, D.; Duarte, C. (2019). "Important contribution of macroalgae to oceanic carbon sequestration". Nature Geoscience. 12: 748–754. doi:10.1038/s41561-019-0421-8. hdl:10754/656768.
- Lehmann, Johannes; Gaunt, John; Rondon, Marco (March 1, 2006). "Bio-char Sequestration in Terrestrial Ecosystems – A Review". Mitigation and Adaptation Strategies for Global Change. 11 (2): 403–427. CiteSeerX 10.1.1.183.1147. doi:10.1007/s11027-005-9006-5. ISSN 1381-2386.
- Nellemann, Christian et al. (2009): Blue Carbon. The Role of Healthy Oceans in Binding Carbon. A Rapid Response Assessment. Arendal, Norway: UNEP/GRID-Arendal
- Macreadie, P.I., Anton, A., Raven, J.A., Beaumont, N., Connolly, R.M., Friess, D.A., Kelleway, J.J., Kennedy, H., Kuwae, T., Lavery, P.S. and Lovelock, C.E. (2019) "The future of Blue Carbon science". Nature communications, 10(1): 1–13. doi:10.1038/s41467-019-11693-w.
- National Academies Of Sciences, Engineering (2019). Negative Emissions Technologies and Reliable Sequestration: A Research Agenda. Washington, D.C.: National Academies of Sciences, Engineering, and Medicine. p. 45. doi:10.17226/25259. ISBN 978-0-309-48452-7. PMID 31120708.
- Ortega, Alejandra; Geraldi, N.R.; Alam, I.; Kamau, A.A.; Acinas, S.; Logares, R.; Gasol, J.; Massana, R.; Krause-Jensen, D.; Duarte, C. (2019). "Important contribution of macroalgae to oceanic carbon sequestration". Nature Geoscience. 12: 748–754. doi:10.1038/s41561-019-0421-8. hdl:10754/656768.
- National Academies of Sciences, Engineering (2019). Negative Emissions Technologies and Reliable Sequestration: A Research Agenda. Washington, DC: National Academies Press. pp. 45–86. doi:10.17226/25259. ISBN 978-0-309-48452-7. PMID 31120708.
- Nelleman, C. "Blue carbon: the role of healthy oceans in binding carbon" (PDF). Archived from the original (PDF) on March 4, 2016.
- National Academies of Sciences, Engineering, and Medicine (2019). "Coastal Blue Carbon". Negative Emissions Technologies and Reliable Sequestration: A Research Agenda. pp. 45–48. doi:10.17226/25259. ISBN 978-0-309-48452-7. PMID 31120708.CS1 maint: multiple names: authors list (link)
- McLeod, E. "A blueprint for blue carbon: toward an improved understanding of the role of vegetated coastal habitats in sequestering CO2" (PDF).
- "Global Status of BECCS Projects 2010". Biorecro AB, Global CCS Institute. 2010. Retrieved January 20, 2012.
- "Global Technology Roadmap for CCS in Industry Biomass-based industrial CO2 sources: biofuels production with CCS" (PDF). ECN. 2011. Retrieved January 20, 2012.
- "First U.S. large demonstration-scale injection of CO2 from a biofuel production facility begins". Retrieved January 20, 2012.
- "Ethanol plant to sequester CO2 emissions". Archived from the original on March 10, 2011. Retrieved January 20, 2012.
- "Production Begins at Biggest Ethanol Plant in Kansas". Retrieved January 20, 2012.
- "The Potential for the Deployment of Negative Emissions Technologies in the UK" (PDF). Grantham Institute for Climate Change, Imperial College. 2010. Retrieved January 16, 2012.
- Archived May 26, 2013, at the Wayback Machine
- "What is biochar?". UK Biochar research center. University of Edinburgh Kings Buildings Edinburgh. Retrieved April 25, 2016.
- "What is Biomass?". Biomass Energy Center. Direct.gov.uk. Archived from the original on October 3, 2016. Retrieved April 25, 2016.
- "Climate change and Biochar". International Biochar Initiative. International Biochar Initiative. Retrieved April 25, 2016.
- "Biochar reducing and removing CO2 while improving soils: A significant sustainable response to climate change" (PDF). UKBRC. UK Biochar research Center. Retrieved April 25, 2016.
- "Maps show rocks ideal for sequestering carbon - NYTimes.com". archive.nytimes.com. Retrieved May 15, 2018.
- U.S. Department of the Interior. "Mapping the Mineral Resource Base for Mineral Carbon-Dioxide Sequestration in the Conterminous United States" (PDF). U.S. Geological Survey. Data Series 414.
- "CarbFix Project | Global Carbon Capture and Storage Institute". www.globalccsinstitute.com. Archived from the original on July 3, 2018. Retrieved May 15, 2018.
- "The CarbFix Project". www.or.is (in Icelandic). August 22, 2017. Retrieved May 15, 2018.
- "Turning Carbon Dioxide Into Rock, and Burying It". The New York Times. February 9, 2015. ISSN 0362-4331. Retrieved May 15, 2018.
- "SAPEA, Science Advice for Policy by European Academies. (2018). Novel carbon capture and utilisation technologies: research and climate aspects Berlin" (PDF). SAPEA. 2018. doi:10.26356/carboncapture.
- Sanz-Pérez, Eloy S.; Murdock, Christopher R.; Didas, Stephanie A.; Jones, Christopher W. (August 25, 2016). "Direct Capture of CO
2 from Ambient Air". Chemical Reviews. 116 (19): 11840–11876. doi:10.1021/acs.chemrev.6b00173. PMID 27560307. - "First Successful Demonstration of Carbon Dioxide Air Capture Technology Achieved by Columbia University Scientist and Private Company". Earth.columbia.edu. Archived from the original on June 22, 2010. Retrieved April 14, 2010.
- Diamandis, Peter H. (August 23, 2019). "The Promise of Direct Air Capture: Making Stuff Out of Thin Air". Singularity Hub. Retrieved August 29, 2019.
- Vidal, John (February 4, 2018). "How Bill Gates aims to clean up the planet". The Observer. ISSN 0029-7712. Retrieved August 26, 2019.
- Service, Robert F. (June 7, 2018). "Cost plunges for capturing carbon dioxide from the air". Science | AAAS. Retrieved August 26, 2019.
- "Direct Air Capture (Technology Factsheet)". Geoengineering Monitor. May 24, 2018. Retrieved August 27, 2019.
- Keith, David W.; Holmes, Geoffrey; St. Angelo, David; Heide, Kenton (June 7, 2018). "A Process for Capturing CO2 from the Atmosphere". Joule. 2 (8): 1573–1594. doi:10.1016/j.joule.2018.05.006.
- "Direct Air Capture (Technology Factsheet)". Geoengineering Monitor. May 24, 2018. Retrieved August 27, 2019.
- "Direct Air Capture of CO2 with Chemicals: A Technology Assessment for the APS Panel on Public Affairs" (PDF). www.aps.org. June 1, 2011. Retrieved August 26, 2019.
- Ranjan, Manya; Herzog, Howard J. (2011). "Feasibility of air capture". Energy Procedia. 4: 2869–2876. doi:10.1016/j.egypro.2011.02.193. ISSN 1876-6102.
- "Novel carbon capture and utilisation technologies: research and climate aspects" (PDF). Science Advice for Policy by European Academies: 50. May 23, 2018. doi:10.26356/carboncapture. ISBN 978-3-9819415-6-2. ISSN 2568-4434.
- NASA Goddard Multimedia Accessed June 2012
- Matear, R. J. & B. Elliott (2004). "Enhancement of oceanic uptake of anthropogenic CO2 by macronutrient fertilization". J. Geophys. Res. 109 (C4): C04001. Bibcode:2004JGRC..10904001M. doi:10.1029/2000JC000321.
- Jones, I.S.F. & Young, H.E. (1997). "Engineering a large sustainable world fishery". Environmental Conservation. 24 (2): 99–104. doi:10.1017/S0376892997000167.
- Keith, David W.; Holmes, Geoffrey; St. Angelo, David; Heidel, Kenton (2018). "A Process for Capturing CO2 from the Atmosphere". Joule. 2 (8): 1573–1594. doi:10.1016/j.joule.2018.05.006.
- "Climate Change Breakthrough: Large-scale capture of atmospheric CO₂ shown to be feasible and affordable". June 7, 2018.
- Köberle, Alexandre C. (December 1, 2019). "The Value of BECCS in IAMs: a Review". Current Sustainable/Renewable Energy Reports. 6 (4): 107–115. doi:10.1007/s40518-019-00142-3. ISSN 2196-3010.
- @elonmusk (January 21, 2021). "Am donating $100M towards a prize for best carbon capture technology" (Tweet) – via Twitter.
- Lackner, Klaus S. (2020). "Practical constraints on atmospheric methane removal". Nature Sustainability. 3 (5): 357–357. doi:10.1038/s41893-020-0496-7. ISSN 2398-9629.
External links
- Krupp, Fred; Keohane, Nathaniel; Pooley, Eric. "Less Than Zero; Can Carbon-Removal Technologies Curb Climate Change?". Foreign Affairs (March/April 2019): 142–152.