Plant microbiome
The plant microbiome plays roles in plant health and productivity and has received significant attention in recent years.[1][2] The microbiome has been defined as "a characteristic microbial community occupying a reasonably well-defined habitat which has distinct physio-chemical properties. The term thus not only refers to the microorganisms involved but also encompasses their theatre of activity".[3][4]
Part of a series on |
Microbiomes |
---|
![]() |
Plants live in association with diverse microbial consortia. These microbes, referred to as the plant's microbiota, live both inside (the endosphere) and outside (the episphere) of plant tissues, and play important roles in the ecology and physiology of plants.[5] "The core plant microbiome is thought to comprise keystone microbial taxa that are important for plant fitness and established through evolutionary mechanisms of selection and enrichment of microbial taxa containing essential functions genes for the fitness of the plant holobiont."[6]
Plant microbiomes are shaped by both factors related to the plant itself, such as genotype, organ, species and health status, as well as factors related to the plant's environment, such as management, land use and climate.[7] The health status of a plant has been reported in some studies to be reflected by or linked to its microbiome.[8][1][9][2]
Overview
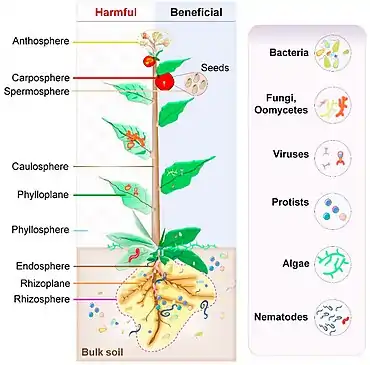
The study of the association of plants with microorganisms precedes that of the animal and human microbiomes, notably the roles of microbes in nitrogen and phosphorus uptake. The most notable examples are plant root-arbuscular mycorrhizal (AM) and legume-rhizobial symbioses, both of which greatly influence the ability of roots to uptake various nutrients from the soil. Some of these microbes cannot survive in the absence of the plant host (obligate symbionts include viruses and some bacteria and fungi), which provides space, oxygen, proteins, and carbohydrates to the microorganisms. The association of AM fungi with plants has been known since 1842, and over 80% of land plants are found associated with them.[11] It is thought AM fungi helped in the domestication of plants.[12][5]
Traditionally, plant-microbe interaction studies have been confined to culturable microbes. The numerous microbes that couldn't be cultured have remained uninvestigated, so knowledge of their roles is largely unknown.[5] The possibilities of unraveling the types and outcomes of these plant-microbe interactions has generated considerable interest among ecologists, evolutionary biologists, plant biologists, and agronomists.[13][14][1] Recent developments in multiomics and the establishment of large collections of microorganisms have dramatically increased knowledge of the plant microbiome composition and diversity. The sequencing of marker genes of entire microbial communities, referred to as metagenomics, sheds light on the phylogenetic diversity of the microbiomes of plants. It also adds to the knowledge of the major biotic and abiotic factors responsible for shaping plant microbiome community assemblages.[14][5]
The focus of plant microbiome studies has been directed at model plants, such as Arabidopsis thaliana, as well as important economic crop species including barley (Hordeum vulgare), corn (Zea mays), rice (Oryza sativa), soybean (Glycine max), wheat (Triticum aestivum), whereas less attention has been given to fruit crops and tree species.[15][2]
The plant microbiota
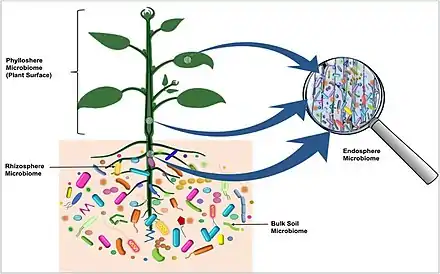
Rhizosphere microbiome
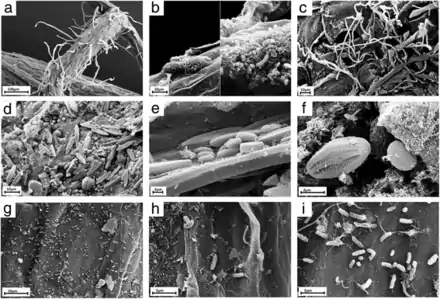
on the roots of Arabidopsis thaliana
a) Overview of an A. thaliana root (primary root) with numerous root hairs. b) Biofilm-forming bacteria. c) Fungal or oomycete hyphae surrounding the root surface. d) Primary root densely covered by spores and protists. e, f) Protists, most likely belonging to the Bacillariophyceae class. g) Bacteria and bacterial filaments. h, i) Different bacterial individuals showing great varieties of shapes and morphological features.[16]
The rhizosphere comprises the 1–10 mm zone of soil immediately surrounding the roots that is under the influence of the plant through its deposition of root exudates, mucilage and dead plant cells.[17] A diverse array of organisms specialize in living in the rhizosphere, including bacteria, fungi, oomycetes, nematodes, algae, protozoa, viruses, and archaea.[18]
The most frequently studied beneficial rhizosphere organisms are mycorrhizae, rhizobium bacteria, plant-growth promoting rhizobacteria (PGPR), and biocontrol microbes. It has been projected that one gram of soil could contain more than one million distinct bacterial genomes,[19] and over 50,000 OTUs (operational taxonomic units) have been found within the potato rhizosphere.[20] Among the prokaryotes in the rhizosphere, the most frequent bacteria are within the Acidobacteria, Proteobacteria, Planctomycetes, Actinobacteria, Bacteroidetes, and Firmicutes.[21][22] In some studies, no significant differences were reported in the microbial community composition between the bulk soil (soil not attached to the plant root) and rhizosphere soil.[23][24] Certain bacterial groups (e. g. Actinobacteria, Xanthomonadaceae) are less abundant in the rhizosphere than in nearby bulk soil .[21][5]
Mycorrhizal fungi are abundant members of the rhizosphere community, and have been found in over 200,000 plant species, and are estimated to associate with over 80% of all plants.[25] Mycorrhizae–root associations play profound roles in land ecosystems by regulating nutrient and carbon cycles. Mycorrhizae are integral to plant health because they provide up to 80% of the nitrogen and phosphorus requirements. In return, the fungi obtain carbohydrates and lipids from host plants.[26] Recent studies of arbuscular mycorrhizal fungi using sequencing technologies show greater between-species and within-species diversity than previously known.[27][5]
– Berendsen et al, 2012 [28]
Phyllosphere microbiome

The aerial surface of a plant (stem, leaf, flower, fruit) is called the phyllosphere and is considered comparatively nutrient poor when compared to the rhizosphere and endosphere. The environment in the phyllosphere is more dynamic than the rhizosphere and endosphere environments. Microbial colonizers are subjected to diurnal and seasonal fluctuations of heat, moisture, and radiation. In addition, these environmental elements affect plant physiology (such as photosynthesis, respiration, water uptake etc.) and indirectly influence microbiome composition.[5] Rain and wind also cause temporal variation to the phyllosphere microbiome.[30]
Overall, there remains high species richness in phyllosphere communities. Fungal communities are highly variable in the phyllosphere of temperate regions and are more diverse than in tropical regions.[31] There can be up to 107 microbes per square centimetre present on the leaf surfaces of plants, and the bacterial population of the phyllosphere on a global scale is estimated to be 1026 cells.[32] The population size of the fungal phyllosphere is likely to be smaller.[33]
Phyllosphere microbes from different plants appear to be somewhat similar at high levels of taxa, but at the lower levels taxa there remain significant differences. This indicates microorganisms may need finely tuned metabolic adjustment to survive in phyllosphere environment.[31] Proteobacteria seems to be the dominant colonizers, with Bacteroidetes and Actinobacteria also predominant in phyllospheres.[34] Although there are similarities between the rhizosphere and soil microbial communities, very little similarity has been found between phyllosphere communities and microorganisms floating in open air (aeroplankton).[35][5]
Endosphere microbiome
Some microorganisms, such as endophytes, penetrate and occupy the plant internal tissues, forming the endospheric microbiome. The arbuscular mycorrhizal and other endophytic fungi are the dominant colonizers of the endosphere.[36] Bacteria, and to some degree archaea, are important members of endosphere communities. Some of these endophytic microbes interact with their host and provide obvious benefits to plants.[21][37][38] Unlike the rhizosphere and the rhizoplane, the endospheres harbor highly specific microbial communities. The root endophytic community can be very distinct from that of the adjacent soil community. In general, diversity of the endophytic community is lower than the diversity of the microbial community outside the plant.[24] The identity and diversity of the endophytic microbiome of above-and below-ground tissues may also differ within the plant.[39][36][5]
The seed microbiome
Individual seeds possessed high microbial diversity, that was higher in the embryo than in the pericarp. Plant seeds can serve as natural vectors for vertical transmission of beneficial endophytes that confer disease resistance.[40] Evidence showing the transmission of microorganisms from seeds to the developing seedling, was found to occur in experimental and natural conditions.[41] It also seems that the transmission to new plant happens through specific mechanisms where certain microorganisms migrate from seed to plant leaves and other to plant roots.[42]
References
- Turner, Thomas R.; James, Euan K.; Poole, Philip S. (2013). "The plant microbiome". Genome Biology. 14 (6): 209. doi:10.1186/gb-2013-14-6-209. PMC 3706808. PMID 23805896.
- Purahong, Witoon; Orrù, Luigi; Donati, Irene; Perpetuini, Giorgia; Cellini, Antonio; Lamontanara, Antonella; Michelotti, Vania; Tacconi, Gianni; Spinelli, Francesco (2018). "Plant Microbiome and Its Link to Plant Health: Host Species, Organs and Pseudomonas syringae pv. Actinidiae Infection Shaping Bacterial Phyllosphere Communities of Kiwifruit Plants". Frontiers in Plant Science. 9: 1563. doi:10.3389/fpls.2018.01563. PMC 6234494. PMID 30464766..
Material was copied from this source, which is available under a Creative Commons Attribution 4.0 International License.
- Whipps J., Lewis K. and Cooke R. (1988) "Mycoparasitism and plant disease control". In: Burge M (Ed.) Fungi in Biological Control Systems, Manchester University Press, pages 161–187. ISBN 9780719019791.
- Berg, Gabriele; Daria Rybakova, Doreen Fischer, Tomislav Cernava, Marie-Christine Champomier Vergès, Trevor Charles, Xiaoyulong Chen, Luca Cocolin, Kellye Eversole, Gema Herrero Corral, Maria Kazou, Linda Kinkel, Lene Lange, Nelson Lima, Alexander Loy, James A. Macklin, Emmanuelle Maguin, Tim Mauchline, Ryan McClure, Birgit Mitter, Matthew Ryan, Inga Sarand, Hauke Smidt, Bettina Schelkle, Hugo Roume, G. Seghal Kiran, Joseph Selvin, Rafael Soares Correa de Souza, Leo van Overbeek, Brajesh K. Singh, Michael Wagner, Aaron Walsh, Angela Sessitsch and Michael Schloter (2020) "Microbiome definition re-visited: old concepts and new challenges". Microbiome, 8(103): 1–22. doi:10.1186/s40168-020-00875-0.
Material was copied from this source, which is available under a Creative Commons Attribution 4.0 International License.
- Dastogeer, K.M., Tumpa, F.H., Sultana, A., Akter, M.A. and Chakraborty, A. (2020) "Plant microbiome–an account of the factors that shape community composition and diversity". Current Plant Biology: 100161. doi:10.1016/j.cpb.2020.100161.
Material was copied from this source, which is available under a Creative Commons Attribution 4.0 International License.
- Compant, S., Samad, A., Faist, H. and Sessitsch, A. (2019) "A review on the plant microbiome: Ecology, functions, and emerging trends in microbial application". Journal of advanced research, 19: 29_37.doi:10.1016/j.jare.2019.03.004.
- Bringel, Franã§Oise; Couã©e, Ivan (2015). "Pivotal roles of phyllosphere microorganisms at the interface between plant functioning and atmospheric trace gas dynamics". Frontiers in Microbiology. 06: 486. doi:10.3389/fmicb.2015.00486. PMC 4440916. PMID 26052316.
- Berendsen, Roeland L.; Pieterse, Corné M.J.; Bakker, Peter A.H.M. (2012). "The rhizosphere microbiome and plant health". Trends in Plant Science. 17 (8): 478–486. doi:10.1016/j.tplants.2012.04.001. hdl:1874/255269. PMID 22564542.
- Berg, Gabriele; Grube, M.; Schloter, M.; Smalla, K. (2014). "The plant microbiome and its importance for plant and human health". Frontiers in Microbiology. 5: 491. doi:10.3389/fmicb.2014.00491. PMC 4166366. PMID 25278934.
- Shelake, R.M., Pramanik, D. and Kim, J.Y. (2019) "Exploration of plant-microbe interactions for sustainable agriculture in CRISPR era". Microorganisms, 7(8): 269. doi:10.3390/microorganisms7080269.
Material was copied from this source, which is available under a Creative Commons Attribution 4.0 International License.
- Koide, Roger T.; Mosse, Barbara (2004). "A history of research on arbuscular mycorrhiza". Mycorrhiza. 14 (3): 145–163. doi:10.1007/s00572-004-0307-4. PMID 15088135. S2CID 1809402.
- Heckman, D. S. (2001). "Molecular Evidence for the Early Colonization of Land by Fungi and Plants". Science. 293 (5532): 1129–1133. doi:10.1126/science.1061457. PMID 11498589. S2CID 10127810.
- Berendsen, Roeland L.; Pieterse, Corné M.J.; Bakker, Peter A.H.M. (2012). "The rhizosphere microbiome and plant health". Trends in Plant Science. 17 (8): 478–486. doi:10.1016/j.tplants.2012.04.001. hdl:1874/255269. PMID 22564542.
- Bulgarelli, Davide; Schlaeppi, Klaus; Spaepen, Stijn; Van Themaat, Emiel Ver Loren; Schulze-Lefert, Paul (2013). "Structure and Functions of the Bacterial Microbiota of Plants". Annual Review of Plant Biology. 64: 807–838. doi:10.1146/annurev-arplant-050312-120106. PMID 23373698.
- Busby, Posy E.; Soman, Chinmay; Wagner, Maggie R.; Friesen, Maren L.; Kremer, James; Bennett, Alison; Morsy, Mustafa; Eisen, Jonathan A.; Leach, Jan E.; Dangl, Jeffery L. (2017). "Research priorities for harnessing plant microbiomes in sustainable agriculture". PLOS Biology. 15 (3): e2001793. doi:10.1371/journal.pbio.2001793. PMC 5370116. PMID 28350798. S2CID 6434145.
- Hassani, M.A., Durán, P. and Hacquard, S. (2018) "Microbial interactions within the plant holobiont". Microbiome, 6(1): 58. doi:10.1186/s40168-018-0445-0.
Material was copied from this source, which is available under a Creative Commons Attribution 4.0 International License.
- Hinsinger, Philippe; Bengough, A. Glyn; Vetterlein, Doris; Young, Iain M. (2009). "Rhizosphere: Biophysics, biogeochemistry and ecological relevance". Plant and Soil. 321 (1–2): 117–152. doi:10.1007/s11104-008-9885-9. S2CID 8997382.
- Bonkowski, Michael; Villenave, Cécile; Griffiths, Bryan (2009). "Rhizosphere fauna: The functional and structural diversity of intimate interactions of soil fauna with plant roots". Plant and Soil. 321 (1–2): 213–233. doi:10.1007/s11104-009-0013-2. S2CID 35701713.
- Gans, J.; Wolinsky, M.; Dunbar, J. (2005). "Computational Improvements Reveal Great Bacterial Diversity and High Metal Toxicity in Soil". Science. 309 (5739): 1387–1390. doi:10.1126/science.1112665. PMID 16123304. S2CID 130269020.
- i̇Nceoğlu, Özgül; Al-Soud, Waleed Abu; Salles, Joana Falcão; Semenov, Alexander V.; Van Elsas, Jan Dirk (2011). "Comparative Analysis of Bacterial Communities in a Potato Field as Determined by Pyrosequencing". PLOS ONE. 6 (8): e23321. doi:10.1371/journal.pone.0023321. PMC 3158761. PMID 21886785.
- Bulgarelli, Davide; Rott, Matthias; Schlaeppi, Klaus; Ver Loren Van Themaat, Emiel; Ahmadinejad, Nahal; Assenza, Federica; Rauf, Philipp; Huettel, Bruno; Reinhardt, Richard; Schmelzer, Elmon; Peplies, Joerg; Gloeckner, Frank Oliver; Amann, Rudolf; Eickhorst, Thilo; Schulze-Lefert, Paul (2012). "Revealing structure and assembly cues for Arabidopsis root-inhabiting bacterial microbiota". Nature. 488 (7409): 91–95. doi:10.1038/nature11336. PMID 22859207. S2CID 4393146.
- Uroz, Stéphane; Buée, Marc; Murat, Claude; Frey-Klett, Pascale; Martin, Francis (2010). "Pyrosequencing reveals a contrasted bacterial diversity between oak rhizosphere and surrounding soil". Environmental Microbiology Reports. 2 (2): 281–288. doi:10.1111/j.1758-2229.2009.00117.x. PMID 23766079.
- Lundberg, Derek S.; Lebeis, Sarah L.; Paredes, Sur Herrera; Yourstone, Scott; Gehring, Jase; Malfatti, Stephanie; Tremblay, Julien; Engelbrektson, Anna; Kunin, Victor; Rio, Tijana Glavina del; Edgar, Robert C.; Eickhorst, Thilo; Ley, Ruth E.; Hugenholtz, Philip; Tringe, Susannah Green; Dangl, Jeffery L. (2012). "Defining the core Arabidopsis thaliana root microbiome". Nature. 488 (7409): 86–90. doi:10.1038/nature11237. PMC 4074413. PMID 22859206.
- Schlaeppi, K.; Dombrowski, N.; Oter, R. G.; Ver Loren Van Themaat, E.; Schulze-Lefert, P. (2014). "Quantitative divergence of the bacterial root microbiota in Arabidopsis thaliana relatives". Proceedings of the National Academy of Sciences. 111 (2): 585–592. doi:10.1073/pnas.1321597111. PMC 3896156. PMID 24379374. S2CID 13806811.
- Van Der Heijden, Marcel G. A.; Martin, Francis M.; Selosse, Marc-André; Sanders, Ian R. (2015). "Mycorrhizal ecology and evolution: The past, the present, and the future". New Phytologist. 205 (4): 1406–1423. doi:10.1111/nph.13288. PMID 25639293.
- Rich, Mélanie K.; Nouri, Eva; Courty, Pierre-Emmanuel; Reinhardt, Didier (2017). "Diet of Arbuscular Mycorrhizal Fungi: Bread and Butter?" (PDF). Trends in Plant Science. 22 (8): 652–660. doi:10.1016/j.tplants.2017.05.008. PMID 28622919.
- Lee, Eun-Hwa; Eo, Ju-Kyeong; Ka, Kang-Hyeon; Eom, Ahn-Heum (2013). "Diversity of Arbuscular Mycorrhizal Fungi and Their Roles in Ecosystems". Mycobiology. 41 (3): 121–125. doi:10.5941/MYCO.2013.41.3.121. PMC 3817225. PMID 24198665.
- Berendsen, R.L., Pieterse, C.M. and Bakker, P.A. (2012) "The rhizosphere microbiome and plant health". Trends in plant science, 17(8): 478–486. doi:10.1016/j.tplants.2012.04.001.
- He, Sheng Yang (2020) When plants and their microbes are not in sync, the results can be disastrous The Conversation, 28 August 2020.
- Lindow, Steven E. (1996). "Role of Immigration and Other Processes in Determining Epiphytic Bacterial Populations". Aerial Plant Surface Microbiology. pp. 155–168. doi:10.1007/978-0-585-34164-4_10. ISBN 978-0-306-45382-3.
- Finkel, Omri M.; Burch, Adrien Y.; Lindow, Steven E.; Post, Anton F.; Belkin, Shimshon (2011). "Geographical Location Determines the Population Structure in Phyllosphere Microbial Communities of a Salt-Excreting Desert Tree". Applied and Environmental Microbiology. 77 (21): 7647–7655. doi:10.1128/AEM.05565-11. PMC 3209174. PMID 21926212.
- Vorholt, Julia A. (2012). "Microbial life in the phyllosphere". Nature Reviews Microbiology. 10 (12): 828–840. doi:10.1038/nrmicro2910. PMID 23154261. S2CID 10447146.
- Lindow, Steven E.; Brandl, Maria T. (2003). "Microbiology of the Phyllosphere". Applied and Environmental Microbiology. 69 (4): 1875–1883. doi:10.1128/AEM.69.4.1875-1883.2003. PMC 154815. PMID 12676659. S2CID 2304379.
- Bodenhausen, Natacha; Horton, Matthew W.; Bergelson, Joy (2013). "Bacterial Communities Associated with the Leaves and the Roots of Arabidopsis thaliana". PLOS ONE. 8 (2): e56329. doi:10.1371/journal.pone.0056329. PMC 3574144. PMID 23457551.
- Vokou, Despoina; Vareli, Katerina; Zarali, Ekaterini; Karamanoli, Katerina; Constantinidou, Helen-Isis A.; Monokrousos, Nikolaos; Halley, John M.; Sainis, Ioannis (2012). "Exploring Biodiversity in the Bacterial Community of the Mediterranean Phyllosphere and its Relationship with Airborne Bacteria". Microbial Ecology. 64 (3): 714–724. doi:10.1007/s00248-012-0053-7. PMID 22544345. S2CID 17291303.
- Vokou, Despoina; Vareli, Katerina; Zarali, Ekaterini; Karamanoli, Katerina; Constantinidou, Helen-Isis A.; Monokrousos, Nikolaos; Halley, John M.; Sainis, Ioannis (2012). "Exploring Biodiversity in the Bacterial Community of the Mediterranean Phyllosphere and its Relationship with Airborne Bacteria". Microbial Ecology. 64 (3): 714–724. doi:10.1007/s00248-012-0053-7. PMID 22544345. S2CID 17291303.
- Dastogeer, Khondoker M.G.; Li, Hua; Sivasithamparam, Krishnapillai; Jones, Michael G.K.; Du, Xin; Ren, Yonglin; Wylie, Stephen J. (2017). "Metabolic responses of endophytic Nicotiana benthamiana plants experiencing water stress". Environmental and Experimental Botany. 143: 59–71. doi:10.1016/j.envexpbot.2017.08.008.
- Rodriguez, R. J.; White Jr, J. F.; Arnold, A. E.; Redman, R. S. (2009). "Fungal endophytes: Diversity and functional roles". New Phytologist. 182 (2): 314–330. doi:10.1111/j.1469-8137.2009.02773.x. PMID 19236579.
- "Experimental Evidence of Microbial Inheritance in Plants and Transmission Routes from Seed to Phyllosphere and Root". 2020-05-14. doi:10.21203/rs.3.rs-27656/v1. Cite journal requires
|journal=
(help) - Matsumoto H, Fan X, Wang Y, Kusstatscher P, Duan J, Wu S, et al. (January 2021). "Bacterial seed endophyte shapes disease resistance in rice". Nature Plants. 7: 60–72. doi:10.1038/s41477-020-00826-5.
- "Seeds transfer their microbes to the next generation". EurekAlert!. Retrieved 2021-01-21.
- Abdelfattah, Ahmed; Wisniewski, Michael; Schena, Leonardo; Tack, Ayco J. M. "Experimental evidence of microbial inheritance in plants and transmission routes from seed to phyllosphere and root". Environmental Microbiology. n/a (n/a). doi:10.1111/1462-2920.15392. ISSN 1462-2920.
Reference books
- Saleem M (2015) Microbiome Community Ecology: Fundamentals and Applications Springer. ISBN 9783319116655.
- Kumar V, Prasad R, Kumar M and Choudhary DK (2019) Microbiome in Plant Health and Disease: Challenges and Opportunities Springer. ISBN 9789811384950.
- Kumar, Vivek; Prasad, Ram; Kumar, Manoj; Choudhary, Devendra K. (10 August 2019). Microbiome in Plant Health and Disease: Challenges and Opportunities. ISBN 9789811384950.
- Grube, Martin; Schloter, Michael; Smalla, Kornelia; Berg, Gabriele (22 January 2015). The plant microbiome and its importance for plant and human health. ISBN 9782889193783. PMID 25278934.
- Egamberdieva, Dilfuza; Ahmad, Parvaiz (6 February 2018). Plant Microbiome: Stress Response. ISBN 9789811055140.
- Castiglione, Stefano; Cicatelli, Angela; Ferrol, Nuria; Rozpadek, Piotr (22 August 2019). Effects of Plant-Microbiome Interactions on Phyto- and Bio-Remediation Capacity. ISBN 9782889459322.
- Castiglione, Stefano; Cicatelli, Angela; Ferrol, Nuria; Rozpadek, Piotr (22 August 2019). Effects of Plant-Microbiome Interactions on Phyto- and Bio-Remediation Capacity. ISBN 9782889459322.
- Carvalhais, Lilia C.; Dennis, Paul G. (30 December 2020). The Plant Microbiome: Methods and Protocols. ISBN 9781071610398.
- Kumar, Vivek; Prasad, Ram; Kumar, Manoj; Choudhary, Devendra K. (10 August 2019). Microbiome in Plant Health and Disease: Challenges and Opportunities. ISBN 9789811384950.
- Solanki, Manoj Kumar; Kashyap, Prem Lal; Ansari, Rizwan Ali; Kumari, Baby (28 August 2020). Microbiomes and Plant Health: Panoply and Their Applications. ISBN 9780128226018.
- Yadav, Ajar Nath (2020). Advances in Plant Microbiome and Sustainable Agriculture: Diversity and Biotechnological Applications. ISBN 9789811532085.
- Varma, Ajit. Plant Microbiome Paradigm. ISBN 9783030503956.
- Doty, Sharon Lafferty (21 September 2017). Functional Importance of the Plant Microbiome: Implications for Agriculture, Forestry and Bioenergy. ISBN 9783319658971.
- Yadav (2020). Advances in Plant Microbiome and Sustainable Agriculture. ISBN 9789811532047.
- Antwis, Rachael E.; Harrison, Xavier A.; Cox, Michael J. (12 March 2020). Microbiomes of Soils, Plants and Animals: An Integrated Approach. ISBN 9781108473712.
- Varma, Ajit; Tripathi, Swati; Prasad, Ram (20 October 2020). Plant Microbiome Paradigm. ISBN 9783030503949.
- Rosenberg, Eugene; Zilber-Rosenberg, Ilana (31 January 2014). The Hologenome Concept: Human, Animal and Plant Microbiota. ISBN 9783319042411.
- Yadav, Ajar Nath; Singh, Joginder; Rastegari, Ali Asghar; Yadav, Neelam (6 March 2020). Plant Microbiomes for Sustainable Agriculture. ISBN 9783030384531.
![]() |
Wikimedia Commons has media related to Plant microbiomes. |