Early Triassic
The Early Triassic is the first of three epochs of the Triassic Period of the geologic timescale. It spans the time between 251.902 Ma and 247.2 Ma (million years ago). Rocks from this epoch are collectively known as the Lower Triassic series, which is a unit in chronostratigraphy.
Early/Lower Triassic | |
---|---|
251.902 ± 0.024 – 247.2 Ma | |
![]() Sandstone from the Lower Triassic Series | |
Chronology | |
Key events in the Triassic -255 — – -250 — – -245 — – -240 — – -235 — – -230 — – -225 — – -220 — – -215 — – -210 — – -205 — – -200 — An approximate timescale of key Triassic events. Axis scale: millions of years ago. (NOTE: The white links are for readability only. They are still clickable.) | |
Etymology | |
Chronostratigraphic name | Lower Triassic |
Geochronological name | Early Triassic |
Name formality | Formal |
Usage information | |
Celestial body | Earth |
Regional usage | Global (ICS) |
Time scale(s) used | ICS Time Scale |
Definition | |
Chronological unit | Epoch |
Stratigraphic unit | Series |
Time span formality | Formal |
Lower boundary definition | FAD of the Conodont Hindeodus parvus |
Lower boundary GSSP | Meishan, Zhejiang, China 31.0798°N 119.7058°E |
GSSP ratified | 2001[5] |
Upper boundary definition | Not formally defined |
Upper boundary definition candidates |
|
Upper boundary GSSP candidate section(s) |
|
The Early Triassic is the oldest epoch of the Mesozoic Era. It is preceded by the Lopingian epoch (late Permian, Paleozoic Era) and followed by the Middle Triassic epoch. The Early Triassic is divided into the Induan and Olenekian ages. The Induan is subdivided into the Griesbachian and Dienerian subages and the Olenekian is subdivided into the Smithian and Spathian subages.[6]
The Lower Triassic series is coeval with the Scythian stage, which is today not included in the official timescales but can be found in older literature. In Europe, most of the Lower Triassic is composed of Buntsandstein, a lithostratigraphic unit of continental red beds.
Early Triassic life
Fauna and Flora
The Permian-Triassic extinction event spawned the Triassic period. The massive extinctions that ended the Permian period and Paleozoic era caused extreme hardships for the surviving species.
The Early Triassic epoch saw the recovery of life after the biggest mass extinction event of the past, which took millions of years due to the severity of the event and the harsh Early Triassic climate.[7] Many types of corals, brachiopods, molluscs, echinoderms, and other invertebrates had disappeared. The Permian vegetation dominated by Glossopteris in the southern hemisphere ceased to exist.[8] Other groups, such as Actinopterygii, appear to have been less affected by this extinction event[9] and body size was not a selective factor during the extinction event.[10][11] Different patterns of recovery are evident on land and in the sea. Early Triassic faunas lacked biodiversity and were relatively homogeneous due to the effects of the extinction. The ecological recovery on land took 30 million years.[12]
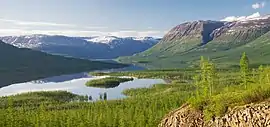
The climate during the Early Triassic epoch (especially in the interior of the supercontinent Pangaea) was generally arid, rainless and dry and deserts were widespread; however the poles possessed a temperate climate. The pole-to-equator temperature gradient was temporally flat during the Early Triassic and may have allowed tropical species to extend their distribution poleward. This is evidenced by the global distribution of ammonoids.[13] The mostly hot climate of the Early Triassic may have been caused by late volcanic eruptions of the Siberian Traps, which had probably triggered the Permian-Triassic extinction event and accelerated the rate of global warming into the Triassic. Studies suggest that Early Triassic climate was volatile, with relatively rapid and large temperature changes.[14][15][16]
Terrestrial Biota
The most common land vertebrate was the small herbivorous synapsid Lystrosaurus. Interpreted as a disaster taxon, Lystrosaurus had a wide range across Pangea. In the southern part of the supercontinent, it co-occurred with the non-mammalian cynodonts Galesaurus and Thrinaxodon, early relatives of mammals. First archosauriforms appeared, such as Erythrosuchus (Olenekian-Ladinian).[17] This group includes the ancestors of crocodiles and dinosaurs (including birds). Fossilized foot prints of dinosauromorphs are known from the Olenekian.[18]
The flora was gymnosperm dominated at the onset of the Triassic, but changed rapidly and became lycopod dominated (e.g. Pleuromeia) during the Griesbachian-Dienerian ecological crisis. This change coincided with the extinction of the Permian Glossopteris flora.[8] In the Spathian subage, the flora changed back to gymnosperm and pteridophyte dominated.[19] These shifts reflect global changes in precipitation and temperature.[8]
Aquatic Biota
In the oceans, the most common Early Triassic hard-shelled marine invertebrates were bivalves, gastropods, ammonites, echinoids, and a few articulate brachiopods. First oysters appeared in the Early Triassic. They grew on the shells of living ammonoids.[20] Microbial reefs were common, possibly due to lack of competition with metazoan reef builders as a result of the extinction.[21] However, transient metazoan reefs reoccurred during the Olenekian wherever permitted by environmental conditions.[22] Ammonoids show blooms followed by extinctions during the Early Triassic.[23]
Aquatic vertebrates diversified after the extinction.
Fishes: Typical Triassic ray-finned fishes, such as Australosomus, Birgeria, Bobasatrania, Boreosomus, Pteronisculus, Parasemionotidae and Saurichthys appeared close to the Permian-Triassic boundary, whereas neopterygians diversified later during the Triassic.[10] Many species of fish had a global distribution during the Early Triassic. Coelacanths show a peak in their diversity and new modes of life (Rebellatrix). Chondrichthyes are represented by Hybodontiformes like Palaeobates, Omanoselache, Lissodus, some Neoselachii, as well as last survivors of Eugeneodontida (Caseodus, Fadenia).
Amphibians: Relatively large, marine temnospondyl amphibians, such as Aphaneramma or Wantzosaurus, were geographically widespread during the Induan and Olenekian ages. The fossils of these crocodile-shaped amphibians were found in Greenland, Spitsbergen, Pakistan and Madagascar.
Reptiles: In the oceans, first marine reptiles appeared during the Early Triassic.[24] Their descendants ruled the oceans during the Mesozoic. Hupehsuchia, Ichthyopterygia and sauropterygians are among the first marine reptiles to enter the scene in the Olenekian (e.g. Cartorhynchus, Chaohusaurus, Utatsusaurus, Hupehsuchus, Grippia, Omphalosaurus, Corosaurus). Other marine reptiles such as Tanystropheus, Helveticosaurus, Atopodentatus, placodonts or the thalattosaurs followed later in the Middle Triassic.[24] The Anisian aged ichthyosaur Thalattoarchon was one of the first marine macropredators capable of eating prey that was similar in size to itself, an ecological role that can be compared to that of modern orcas.[25]
Smithian-Spathian boundary extinction
An important extinction event occurred during the Olenekian age of the Early Triassic, near the Smithian and Spathian subage boundary. The main victims of this Smithian-Spathian boundary extinction event[26] were ammonoids and conodonts, as well as a few Palaeozoic species that survived the Permian-Triassic extinction event. Marine reptiles, such as ichthyopterygians and sauropterygians, diversified after the extinction.
The flora was also affected significantly. It changed from lycopod dominated (e.g. Pleuromeia) during the Dienerian and Smithian subages to gymnosperm and pteridophyte dominated in the Spathian.[27][28] These vegetation changes are due to global changes in temperature and precipitation. Conifers (gymnosperms) were the dominant plants during most of the Mesozoic. Until recently the existence of this extinction event about 249.4 Ma ago[29] was not recognised.[30]
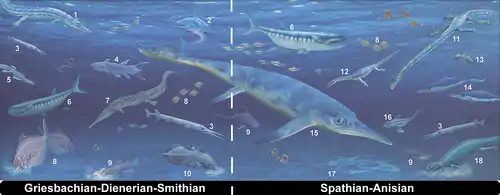
The Smithian-Spathian boundary extinction was linked to late eruptions of the Siberian Traps, which resulted in climate change.[14] Oxygen isotope studies on conodonts have revealed that temperatures rose in the first 2 million years of the Triassic, ultimately reaching sea surface temperatures of up to 40 °C (104 °F) in the tropics during the Smithian.[31] The extinction itself occurred during a subsequent drop in global temperatures in the latest Smithian; however, temperature alone cannot account for the Smithian-Spathian boundary extinction, because several factors were at play.[16][32]
In the ocean, many large and mobile species moved away from the tropics, but large fish remained,[33] and amongst the immobile species such as molluscs, only the ones that could cope with the heat survived; half the bivalves disappeared.[34] On land, the tropics were nearly devoid of life.[15] Many big, active animals only returned to the tropics, and plants recolonised on land when temperatures returned to normal.
There is evidence that life had recovered rapidly, at least locally. This is indicated by sites that show exceptionally high biodiversity (e.g. the earliest Spathian Paris Biota),[35] which suggest that food webs were complex and comprised several trophic levels.
Fossil gallery
- Early Triassic brittle stars (echinoderms)
- Early Triassic ammonoid Hedenstroemia
- Fossil of the Early Triassic neopterygian Candelarialepis argentus
- Early Triassic Hupehsuchus fossil in the Paleozoological Museum of China
- Skull of the Early Triassic archosauriform Erythrosuchus
- Lystrosaurus hedini skeleton at the Museum of Paleontology in Tübingen
See also
References
- Widmann, Philipp; Bucher, Hugo; Leu, Marc; Vennemann, Torsten; Bagherpour, Borhan; Schneebeli-Hermann, Elke; Goudemand, Nicolas; Schaltegger, Urs (2020). "Dynamics of the Largest Carbon Isotope Excursion During the Early Triassic Biotic Recovery". Frontiers in Earth Science. 8 (196): 1–16. doi:10.3389/feart.2020.00196.
- McElwain, J. C.; Punyasena, S. W. (2007). "Mass extinction events and the plant fossil record". Trends in Ecology & Evolution. 22 (10): 548–557. doi:10.1016/j.tree.2007.09.003. PMID 17919771.
- |note5-nudge-down=1 Retallack, G. J.; Veevers, J.; Morante, R. (1996). "Global coal gap between Permian–Triassic extinctions and middle Triassic recovery of peat forming plants". GSA Bulletin. 108 (2): 195–207. doi:10.1130/0016-7606(1996)108<0195:GCGBPT>2.3.CO;2. Retrieved 2007-09-29.
- Payne, J. L.; Lehrmann, D. J.; Wei, J.; Orchard, M. J.; Schrag, D. P.; Knoll, A. H. (2004). "Large Perturbations of the Carbon Cycle During Recovery from the End-Permian Extinction". Science. 305 (5683): 506–9. doi:10.1126/science.1097023. PMID 15273391.
- Hongfu, Yin; Kexin, Zhang; Jinnan, Tong; Zunyi, Yang; Shunbao, Wu (June 2001). "The Global Stratotype Section and Point (GSSP) of the Permian-Triassic Boundary" (PDF). Episodes. 24 (2): 102–114. doi:10.18814/epiiugs/2001/v24i2/004. Retrieved 8 December 2020.
- Tozer, Edward T. (1965). Lower Triassic stages and ammonoid zones of arctic Canada. Geological Survey of Canada. OCLC 606894884.
- Chen, Zhong-Qiang; Benton, Michael J. (27 May 2012). "The timing and pattern of biotic recovery following the end-Permian mass extinction". Nature Geoscience. 5 (6): 375–383. Bibcode:2012NatGe...5..375C. doi:10.1038/ngeo1475.
- Hochuli, Peter A.; Sanson-Barrera, Anna; Schneebeli-Hermann, Elke; Bucher, Hugo (24 June 2016). "Severest crisis overlooked—Worst disruption of terrestrial environments postdates the Permian–Triassic mass extinction". Scientific Reports. 6 (1): 28372. Bibcode:2016NatSR...628372H. doi:10.1038/srep28372. PMC 4920029. PMID 27340926.
- Smithwick, Fiann M.; Stubbs, Thomas L. (2 February 2018). "Phanerozoic survivors: Actinopterygian evolution through the Permo‐Triassic and Triassic‐Jurassic mass extinction events". Evolution. 72 (2): 348–362. doi:10.1111/evo.13421. PMC 5817399. PMID 29315531.
- Romano, Carlo; Koot, Martha B.; Kogan, Ilja; Brayard, Arnaud; Minikh, Alla V.; Brinkmann, Winand; Bucher, Hugo; Kriwet, Jürgen (February 2016). "Permian-Triassic Osteichthyes (bony fishes): diversity dynamics and body size evolution". Biological Reviews. 91 (1): 106–147. doi:10.1111/brv.12161. PMID 25431138. S2CID 5332637.
- Puttick, Mark N.; Kriwet, Jürgen; Wen, Wen; Hu, Shixue; Thomas, Gavin H.; Benton, Michael J.; Angielczyk, Kenneth (September 2017). "Body length of bony fishes was not a selective factor during the biggest mass extinction of all time". Palaeontology. 60 (5): 727–741. doi:10.1111/pala.12309.
- Sahney, Sarda; Benton, Michael J (15 January 2008). "Recovery from the most profound mass extinction of all time". Proceedings of the Royal Society B: Biological Sciences. 275 (1636): 759–765. doi:10.1098/rspb.2007.1370. PMC 2596898. PMID 18198148.
- Brayard, Arnaud; Bucher, Hugo; Escarguel, Gilles; Fluteau, Frédéric; Bourquin, Sylvie; Galfetti, Thomas (September 2006). "The Early Triassic ammonoid recovery: Paleoclimatic significance of diversity gradients". Palaeogeography, Palaeoclimatology, Palaeoecology. 239 (3–4): 374–395. Bibcode:2006PPP...239..374B. doi:10.1016/j.palaeo.2006.02.003.
- Romano, Carlo; Goudemand, Nicolas; Vennemann, Torsten W.; Ware, David; Schneebeli-Hermann, Elke; Hochuli, Peter A.; Brühwiler, Thomas; Brinkmann, Winand; Bucher, Hugo (21 December 2012). "Climatic and biotic upheavals following the end-Permian mass extinction". Nature Geoscience. 6 (1): 57–60. doi:10.1038/ngeo1667. S2CID 129296231.
- Sun, Y.; Joachimski, M. M.; Wignall, P. B.; Yan, C.; Chen, Y.; Jiang, H.; Wang, L.; Lai, X. (18 October 2012). "Lethally Hot Temperatures During the Early Triassic Greenhouse". Science. 338 (6105): 366–370. Bibcode:2012Sci...338..366S. doi:10.1126/science.1224126. PMID 23087244. S2CID 41302171.
- Goudemand, Nicolas; Romano, Carlo; Leu, Marc; Bucher, Hugo; Trotter, Julie A.; Williams, Ian S. (August 2019). "Dynamic interplay between climate and marine biodiversity upheavals during the early Triassic Smithian -Spathian biotic crisis". Earth-Science Reviews. 195: 169–178. Bibcode:2019ESRv..195..169G. doi:10.1016/j.earscirev.2019.01.013.
- Foth, Christian; Ezcurra, Martín D.; Sookias, Roland B.; Brusatte, Stephen L.; Butler, Richard J. (15 September 2016). "Unappreciated diversification of stem archosaurs during the Middle Triassic predated the dominance of dinosaurs". BMC Evolutionary Biology. 16 (1): 188. doi:10.1186/s12862-016-0761-6. PMC 5024528. PMID 27628503.
- Brusatte, Stephen L.; Niedźwiedzki, Grzegorz; Butler, Richard J. (6 October 2010). "Footprints pull origin and diversification of dinosaur stem lineage deep into Early Triassic". Proceedings of the Royal Society B: Biological Sciences. 278 (1708): 1107–1113. doi:10.1098/rspb.2010.1746. PMC 3049033. PMID 20926435.
- Schneebeli-Hermann, Elke; Kürschner, Wolfram M.; Kerp, Hans; Bomfleur, Benjamin; Hochuli, Peter A.; Bucher, Hugo; Ware, David; Roohi, Ghazala (April 2015). "Vegetation history across the Permian–Triassic boundary in Pakistan (Amb section, Salt Range)". Gondwana Research. 27 (3): 911–924. Bibcode:2015GondR..27..911S. doi:10.1016/j.gr.2013.11.007.
- Hautmann, Michael; Ware, David; Bucher, Hugo (August 2017). "Geologically oldest oysters were epizoans on Early Triassic ammonoids". Journal of Molluscan Studies. 83 (3): 253–260. doi:10.1093/mollus/eyx018.
- Foster, William J.; Heindel, Katrin; Richoz, Sylvain; Gliwa, Jana; Lehrmann, Daniel J.; Baud, Aymon; Kolar‐Jurkovšek, Tea; Aljinović, Dunja; Jurkovšek, Bogdan; Korn, Dieter; Martindale, Rowan C.; Peckmann, Jörn (20 November 2019). "Suppressed competitive exclusion enabled the proliferation of Permian/Triassic boundary microbialites". The Depositional Record. 6 (1): 62–74. doi:10.1002/dep2.97. PMC 7043383. PMID 32140241.
- Brayard, Arnaud; Vennin, Emmanuelle; Olivier, Nicolas; Bylund, Kevin G.; Jenks, Jim; Stephen, Daniel A.; Bucher, Hugo; Hofmann, Richard; Goudemand, Nicolas; Escarguel, Gilles (18 September 2011). "Transient metazoan reefs in the aftermath of the end-Permian mass extinction". Nature Geoscience. 4 (10): 693–697. Bibcode:2011NatGe...4..693B. doi:10.1038/ngeo1264.
- Brayard, A.; Escarguel, G.; Bucher, H.; Monnet, C.; Bruhwiler, T.; Goudemand, N.; Galfetti, T.; Guex, J. (27 August 2009). "Good Genes and Good Luck: Ammonoid Diversity and the End-Permian Mass Extinction". Science. 325 (5944): 1118–1121. Bibcode:2009Sci...325.1118B. doi:10.1126/science.1174638. PMID 19713525. S2CID 1287762.
- Scheyer, Torsten M.; Romano, Carlo; Jenks, Jim; Bucher, Hugo (19 March 2014). "Early Triassic Marine Biotic Recovery: The Predators' Perspective". PLOS ONE. 9 (3): e88987. Bibcode:2014PLoSO...988987S. doi:10.1371/journal.pone.0088987. PMC 3960099. PMID 24647136.
- Fröbisch, Nadia B.; Fröbisch, Jörg; Sander, P. Martin; Schmitz, Lars; Rieppel, Olivier (22 January 2013). "Macropredatory ichthyosaur from the Middle Triassic and the origin of modern trophic networks". Proceedings of the National Academy of Sciences. 110 (4): 1393–1397. Bibcode:2013PNAS..110.1393F. doi:10.1073/pnas.1216750110. PMC 3557033. PMID 23297200.
- Galfetti, Thomas; Hochuli, Peter A.; Brayard, Arnaud; Bucher, Hugo; Weissert, Helmut; Vigran, Jorunn Os (2007). "Smithian-Spathian boundary event: Evidence for global climatic change in the wake of the end-Permian biotic crisis". Geology. 35 (4): 291. Bibcode:2007Geo....35..291G. doi:10.1130/G23117A.1.
- Schneebeli-Hermann, Elke; Kürschner, Wolfram M.; Kerp, Hans; Bomfleur, Benjamin; Hochuli, Peter A.; Bucher, Hugo; Ware, David; Roohi, Ghazala (April 2015). "Vegetation history across the Permian–Triassic boundary in Pakistan (Amb section, Salt Range)". Gondwana Research. 27 (3): 911–924. Bibcode:2015GondR..27..911S. doi:10.1016/j.gr.2013.11.007.
- Goudemand, Nicolas; Romano, Carlo; Leu, Marc; Bucher, Hugo; Trotter, Julie A.; Williams, Ian S. (August 2019). "Dynamic interplay between climate and marine biodiversity upheavals during the early Triassic Smithian -Spathian biotic crisis". Earth-Science Reviews. 195: 169–178. Bibcode:2019ESRv..195..169G. doi:10.1016/j.earscirev.2019.01.013.
- Widmann, Philipp; Bucher, Hugo; Leu, Marc; Vennemann, Torsten; Bagherpour, Borhan; Schneebeli-Hermann, Elke; Goudemand, Nicolas; Schaltegger, Urs (2020). "Dynamics of the Largest Carbon Isotope Excursion During the Early Triassic Biotic Recovery". Frontiers in Earth Science. 8 (196): 1–16. doi:10.3389/feart.2020.00196.
- Hallam, A.; Wignall, P. B. (1997). Mass Extinctions and Their Aftermath. Oxford University Press, UK. p. 143. ISBN 978-0-19-158839-6.
Extinctions with and at the close of the Triassic
- Marshall, Michael (18 October 2012). "Roasting Triassic heat exterminated tropical life". New Scientist.
- Widmann, Philipp; Bucher, Hugo; Leu, Marc; Vennemann, Torsten; Bagherpour, Borhan; Schneebeli-Hermann, Elke; Goudemand, Nicolas; Schaltegger, Urs (2020). "Dynamics of the Largest Carbon Isotope Excursion During the Early Triassic Biotic Recovery". Frontiers in Earth Science. 8 (196): 1–16. doi:10.3389/feart.2020.00196.
- Romano, Carlo; Jenks, James F.; Jattiot, Romain; Scheyer, Torsten M.; Bylund, Kevin G.; Bucher, Hugo (19 July 2017). "Marine Early Triassic Actinopterygii from Elko County (Nevada, USA): implications for the Smithian equatorial vertebrate eclipse". Journal of Paleontology. 91 (5): 1025–1046. doi:10.1017/jpa.2017.36.
- Hallam, A.; Wignall, P. B. (1997). Mass Extinctions and Their Aftermath. Oxford University Press, UK. p. 144. ISBN 978-0-19-158839-6.
- Brayard, Arnaud; Krumenacker, L. J.; Botting, Joseph P.; Jenks, James F.; Bylund, Kevin G.; Fara, Emmanuel; Vennin, Emmanuelle; Olivier, Nicolas; Goudemand, Nicolas; Saucède, Thomas; Charbonnier, Sylvain; Romano, Carlo; Doguzhaeva, Larisa; Thuy, Ben; Hautmann, Michael; Stephen, Daniel A.; Thomazo, Christophe; Escarguel, Gilles (15 February 2017). "Unexpected Early Triassic marine ecosystem and the rise of the Modern evolutionary fauna". Science Advances. 3 (2): e1602159. Bibcode:2017SciA....3E2159B. doi:10.1126/sciadv.1602159. PMC 5310825. PMID 28246643.
Further reading
- Martinetto, Edoardo; Tschopp, Emanuel; Gastaldo, Robert, eds. (2020). Nature through Time: Virtual field trips through the Nature of the past. Springer International Publishing. ISBN 978-3-030-35057-4.