Praseodymium(III,IV) oxide
Praseodymium (III,IV) oxide is the inorganic compound with the formula Pr6O11 that is insoluble in water.[2] It has a cubic fluorite structure.[3] It is the most stable form of praseodymium oxide at ambient temperature and pressure.[4]
Names | |
---|---|
IUPAC name
Praseodymium (III,IV) oxide | |
Identifiers | |
3D model (JSmol) |
|
ECHA InfoCard | 100.031.676 ![]() |
EC Number |
|
PubChem CID |
|
CompTox Dashboard (EPA) |
|
| |
| |
Properties | |
Pr6O11 | |
Molar mass | 1021.44 g/mol |
Appearance | dark brown powder |
Density | 6.5 g/mL |
Melting point | 2,183 °C (3,961 °F; 2,456 K).[1] |
Boiling point | 3,760 °C (6,800 °F; 4,030 K)[1] |
Hazards | |
GHS pictograms | ![]() |
GHS Signal word | Warning |
H315, H319, H335 | |
P261, P305+351+338 | |
Lethal dose or concentration (LD, LC): | |
LD50 (median dose) |
5000 mg·kg−1 Rat oral |
Except where otherwise noted, data are given for materials in their standard state (at 25 °C [77 °F], 100 kPa). | |
Infobox references | |
Properties and structure
Pr6O11 adopts a cubic fluorite crystal structure, measured by XRD, TEM and SEM methods.[3][5] It can be considered an oxygen deficient form of praseodymium (IV) oxide (PrO2), with the Pr ions being in a mixed valency state Pr(III) and Pr(IV).[5] This characteristic is what gives the oxide its many useful properties for its catalytic activity.
Synthesis
Praseodymium oxide nanoparticles are generally produced via solid-state methods such as thermolysis, molten salt method, calcination or precipitation.[3][4] Practically all processes, however, contain a calcination step in order to obtain a crystalline Pr6O11 nanoparticles.
Calcination
Typically, praseodymium nitrate Pr(NO3)3·6H2O[3][5] or praseodymium hydroxide Pr(OH)3[6] is heated at high temperatures (usually above 500 °C) under air to give praseodymium (III,IV) oxide. While less common, synthesis from other organic precursors such as praseodymium acetate, oxalate[7] and malonate[8] have also been reported in literature.
The physical properties of the prepared nanoparticles such as particle shape or lattice parameter depend strongly on the conditions of calcination, such as the temperature or duration, as well as the different preparation methods (calcination, sol-gel, precipitation, for example). As a result, many synthesis routes have been explored to obtain the precise morphology desired.[3][4][5]
Uses
Praseodymium (III,IV) oxide has a number of potential applications in chemical catalysis, and is often used in conjunction with a promoter such as sodium or gold to improve its catalytic performance.
Oxidative coupling of methane
Sodium or lithium promoted praseodymium (III,IV) oxide displays good conversion rate of methane with a good selectivity towards ethane and ethene as opposed to unwanted byproducts such as carbon dioxide.[9][10] While the precise mechanism for this reaction is still under debate, it has been proposed that typically, methane is activated to a methyl radical by oxygen on the surface of the catalyst which combines to form ethane. Ethene is then formed by reduction of ethane either by the catalyst or spontaneously. The multiple oxidation states of Pr(III) and Pr(IV) allows rapid regeneration of the active catalyst species involving a peroxide anion O22-.[9]
This reaction is of particular interest as it enables the conversion of abundant methane gas (composing up to 60% of natural gas)[9][10] into higher order hydrocarbons, which provide more applications. As a result, the oxidative coupling of methane is an economically desirable process.
CO oxidation
In the proposed mechanism for Pr6O11-catalysed oxidation of CO to CO2, CO first binds to the catalyst surface to create a bidentate carbonate then converted to a monodentate carbonate species which can now decompose as CO2, completing the catalyst cycle. The conversion of a bidentate carbonate to a monodentate species leaves an oxygen vacancy on the catalyst surface which can quickly be filled due to the high oxygen mobility deriving from the mixed oxidation states of Pr centres. This proposed mechanism is presented schematically below, adapted from Borchert, et. al.[5]
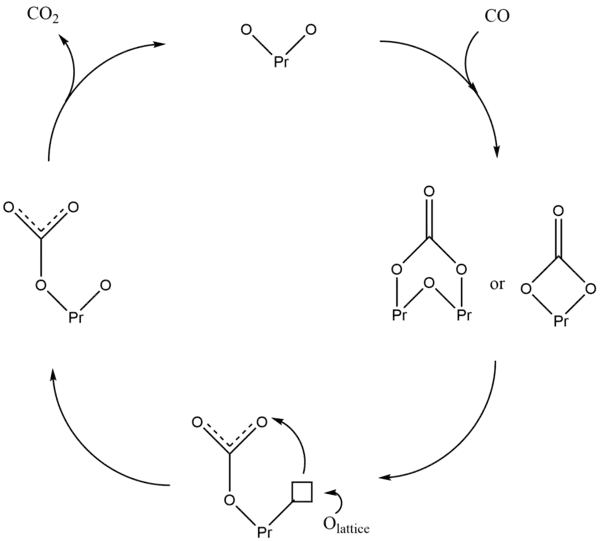
Addition of gold promoters to the catalyst may significantly lower the reaction temperature from 550 °C to 140 °C, but the mechanism is yet to be discovered. It is believed that there is a certain synergistic effect between gold and praseodymium (III,IV) oxide species.[11]
The interest in CO oxidation lies in its ability to convert toxic CO gas to non-toxic CO2 and has applications in car exhaust, for example, which emits CO.[12]
Pr6O11 is also used in conjunction with other additives such as silica or zircon to produce pigments for use in ceramics and glass[13]
References
- "Praseodymium Oxide Nanoparticles (Pr6O11) – Properties, Applications". AZoNano.com. 2013-04-17. Retrieved 2018-03-15.
- "Praseodymium Oxide (Pr6O11)". www.reade.com. Retrieved 2018-03-15.
- Matović, Branko; Pantić, Jelena; Prekajski, Marija; Stanković, Nadežda; Bučevac, Dušan; Minović, Tamara; Čebela, Maria (2013). "Synthesis and characterization of Pr6O11 nanopowders". Ceramics International. 39 (3): 3151–3155. doi:10.1016/j.ceramint.2012.09.098.
- Zinatloo-Ajabshir, Sahar; Salavati-Niasari, Masoud (2015). "Novel poly(ethyleneglycol)-assisted synthesis of praseodymium oxide nanostructures via a facile precipitation route". Ceramics International. 41 (1): 567–575. doi:10.1016/j.ceramint.2014.08.105.
- Borchert, Yulia; Sonström, Patrick; Wilhelm, Michaela; Borchert, Holger; Bäumer, Marcus (2008). "Nanostructured Praseodymium Oxide: Preparation, Structure, and Catalytic Properties". The Journal of Physical Chemistry C. 112 (8): 3054–3063. doi:10.1021/jp0768524. ISSN 1932-7447.
- Ma, Lin; Chen, Weixiang; Zhao, Jie; Zheng, Yifeng; Li, Xiang; Xu, Zhude (2007). "Microwave-assisted synthesis of praseodymium hydroxide nanorods and thermal conversion to oxide nanorod". Materials Letters. 61 (8–9): 1711–1714. doi:10.1016/j.matlet.2006.07.116.
- Hussein, Gamal A.M. (1994). "Formation of praseodymium oxide from the thermal decomposition of hydrated praseodymium acetate and oxalate". Journal of Analytical and Applied Pyrolysis. 29 (1): 89–102. doi:10.1016/0165-2370(93)00782-i.
- Muraishi, Kazuo; Yokobayashi, Hiroko; Nagase, Kenzo (1991). "Systematics on the thermal reactions of lanthanide malonates Ln2(C3H2O4)3 · nH2O in the solid state". Thermochimica Acta. 182 (2): 209–217. doi:10.1016/0040-6031(91)80006-5.
- GAFFNEY, A (1988). "Oxidative coupling of methane over sodium promoted praseodymium oxide". Journal of Catalysis. 114 (2): 422–432. doi:10.1016/0021-9517(88)90045-0.
- Poirier, Michel G.; Breault, Raymond; Kaliaguine, Serge; Adnot, Alain (1991). "Oxidative coupling of methane over praseodymium oxide catalysts". Applied Catalysis. 71 (1): 103–122. doi:10.1016/0166-9834(91)85009-k.
- Huang, P. X.; Wu, F.; Zhu, B. L.; Li, G. R.; Wang, Y. L.; Gao, X. P.; Zhu, H. Y.; Yan, T. Y.; Huang, W. P. (2006-02-01). "Praseodymium Hydroxide and Oxide Nanorods and Au/Pr6O11 Nanorod Catalysts for CO Oxidation". The Journal of Physical Chemistry B. 110 (4): 1614–1620. doi:10.1021/jp055622r. ISSN 1520-6106. PMID 16471724.
- Kim, Il Hee; Seo, Hyun Ook; Park, Eun Ji; Han, Sang Wook; Kim, Young Dok (2017-01-16). "Low Temperature CO oxidation over Iron Oxide Nanoparticles Decorating Internal Structures of a Mesoporous Alumina". Scientific Reports. 7: 40497. Bibcode:2017NatSR...740497K. doi:10.1038/srep40497. ISSN 2045-2322. PMC 5238452. PMID 28091561.
- Kar, J. K; Stevens, R.; Bowen, C. R (2005). "Processing and characterisation of Pr–zircon pigment powder". Advances in Applied Ceramics. 104 (5): 233–238. doi:10.1179/174367605X16699. S2CID 55057492.