Oxide
An oxide (/ˈɒksaɪd/) is a chemical compound that contains at least one oxygen atom and one other element[1] in its chemical formula. "Oxide" itself is the dianion of oxygen, an O2– (molecular) ion. Metal oxides thus typically contain an anion of oxygen in the oxidation state of −2. Most of the Earth's crust consists of solid oxides, the result of elements being oxidized by the oxygen in air or in water. Even materials considered pure elements often develop an oxide coating. For example, aluminium foil develops a thin skin of Al2O3 (called a passivation layer) that protects the foil from further corrosion.[2] Certain elements can form multiple oxides, differing in the amounts of the element combining with the oxygen. Examples are carbon, iron, nitrogen (see nitrogen oxide), silicon, titanium, and aluminium. In such cases the oxides are distinguished by specifying the numbers of atoms involved, as in carbon monoxide and carbon dioxide, or by specifying the element's oxidation number, as in iron(II) oxide and iron(III) oxide.
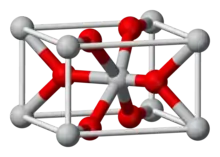
Formation
Due to its electronegativity, oxygen forms stable chemical bonds with almost all elements to give the corresponding oxides. Noble metals (such as gold or platinum) are prized because they resist direct chemical combination with oxygen, and substances like gold(III) oxide must be generated by indirect routes.
Two independent pathways for corrosion of elements are hydrolysis and oxidation by oxygen. The combination of water and oxygen is even more corrosive. Virtually all elements burn in an atmosphere of oxygen or an oxygen-rich environment. In the presence of water and oxygen (or simply air), some elements— sodium—react rapidly, to give the hydroxides. In part, for this reason, alkali and alkaline earth metals are not found in nature in their metallic, i.e., native, form. Cesium is so reactive with oxygen that it is used as a getter in vacuum tubes, and solutions of potassium and sodium, so-called NaK are used to deoxygenate and dehydrate some organic solvents. The surface of most metals consists of oxides and hydroxides in the presence of air. A well-known example is aluminium foil, which is coated with a thin film of aluminium oxide that passivates the metal, slowing further corrosion. The aluminum oxide layer can be built to greater thickness by the process of electrolytic anodizing. Though solid magnesium and aluminum react slowly with oxygen at STP—they, like most metals, burn in air, generating very high temperatures. Finely grained powders of most metals can be dangerously explosive in air. Consequently, they are often used in solid-fuel rockets.
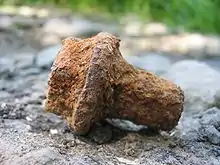
In dry oxygen, iron readily forms iron(II) oxide, but the formation of the hydrated ferric oxides, Fe2O3−x(OH)2x, that mainly comprise rust, typically requires oxygen and water. Free oxygen production by photosynthetic bacteria some 3.5 billion years ago precipitated iron out of solution in the oceans as Fe2O3 in the economically important iron ore hematite.
Structure
Oxides have a range of different structures, from individual molecules to polymeric and crystalline structures. At standard conditions, oxides may range from solids to gases.
Oxides of metals
Oxides of most metals adopt polymeric structures.[3] The oxide typically links three metal atoms (e.g., rutile structure) or six metal atoms (carborundum or rock salt structures). Because the M-O bonds are typically strong and these compounds are crosslinked polymers, the solids tend to be insoluble in solvents, though they are attacked by acids and bases. The formulas are often deceptively simple where many are nonstoichiometric compounds.[2]
Molecular oxides
- Some important gaseous oxides
- Carbon dioxide is the main product of fossil fuel combustion.
- Carbon monoxide is the product of the incomplete combustion of carbon-based fuels and a precursor to many useful chemicals.
- Nitrogen dioxide is a problematic pollutant from internal combustion engines.
- Sulfur dioxide, the principal oxide of sulfur, is emitted from volcanoes.
- Nitrous oxide ("laughing gas") is a potent greenhouse gas produced by soil bacteria.
Although most metal oxides are polymeric, some oxides are molecules. Examples of molecular oxides are carbon dioxide and carbon monoxide. All simple oxides of nitrogen are molecular, e.g., NO, N2O, NO2 and N2O4. Phosphorus pentoxide is a more complex molecular oxide with a deceptive name, the real formula being P4O10. Some polymeric oxides depolymerize when heated to give molecules, examples being selenium dioxide and sulfur trioxide. Tetroxides are rare. The more common examples: ruthenium tetroxide, osmium tetroxide, and xenon tetroxide.
Many oxyanions are known, such as polyphosphates and polyoxometalates. Oxycations are rarer, some examples being nitrosonium (NO+), vanadyl (VO2+), and uranyl (UO2+
2). Of course many compounds are known with both oxides and other groups. In organic chemistry, these include ketones and many related carbonyl compounds. For the transition metals, many oxo complexes are known as well as oxyhalides.
Reduction
Conversion of a metal oxide to the metal is called reduction. The reduction can be induced by many reagents. Many metal oxides convert to metals simply by heating.
Reduction by carbon
Metals are "won" from their oxides by chemical reduction, i.e. by the addition of a chemical reagent. A common and cheap reducing agent is carbon in the form of coke. The most prominent example is that of iron ore smelting. Many reactions are involved, but the simplified equation is usually shown as:[2]
- 2 Fe2O3 + 3 C → 4 Fe + 3 CO2
Metal oxides can be reduced by organic compounds. This redox process is the basis for many important transformations in chemistry, such as the detoxification of drugs by the P450 enzymes and the production of ethylene oxide, which is converted to antifreeze. In such systems, the metal center transfers an oxide ligand to the organic compound followed by regeneration of the metal oxide, often by oxygen in the air.
Reduction by heating
Metals that are lower in the reactivity series can be reduced by heating alone. For example, silver oxide decomposes at 200 °C:[4]
- 2 Ag2O → 4 Ag + O2
Reduction by displacement
Metals that are more reactive displace the oxide of the metals that are less reactive. For example, zinc is more reactive than copper, so it displaces copper (II) oxide to form zinc oxide:
- Zn + CuO → ZnO + Cu
Reduction by hydrogen
Apart from metals, hydrogen can also displace metal oxides to form hydrogen oxide, also known as water:
- H2 + CuO → Cu + H2O
Reduction by electrolysis
Since metals that are reactive form oxides that are stable, some metal oxides must be electrolyzed to be reduced. This includes sodium oxide, potassium oxide, calcium oxide, magnesium oxide, and aluminium oxide. The oxides must be molten before immersing graphite electrodes in them:
- 2Al2O3 → 4Al + 3O2
Hydrolysis and dissolution
Oxides typically react with acids or bases, sometimes both. Those reacting only with acids are labeled basic oxides. Those reacting only by bases are called "acidic oxides". Oxides that react with both are amphoteric. Metals tend to form basic oxides, non-metals tend to form acidic oxides, and amphoteric oxides are formed by elements near the boundary between metals and non-metals (metalloids). This reactivity is the basis of many practical processes, such as the extraction of some metals from their ores in the process called hydrometallurgy.
Oxides of more electropositive elements tend to be basic. They are called basic anhydrides. Exposed to water, they may form basic hydroxides. For example, sodium oxide is basic—when hydrated, it forms sodium hydroxide. Oxides of more electronegative elements tend to be acidic. They are called "acid anhydrides"; adding water, they form oxoacids. For example, dichlorine heptoxide is an acid anhydride; perchloric acid is its fully hydrated form. Some oxides can act as both acid and base. They are amphoteric. An example is aluminium oxide. Some oxides do not show behavior as either acid or base.
The oxide ion has the formula O2−. It is the conjugate base of the hydroxide ion, OH− and is encountered in ionic solids such as calcium oxide. O2− is unstable in aqueous solution − its affinity for H+ is so great (pKb ~ −38) that it abstracts a proton from a solvent H2O molecule:
- O2− + H2O → 2 OH−
The equilibrium constant of aforesaid reactions is pKeq ~ −22
In the 18th century, oxides were named calxes or calces after the calcination process used to produce oxides. Calx was later replaced by oxyd.
Reductive dissolution
The reductive dissolution of a transition metal oxide occurs when dissolution is coupled to a redox event.[5] For example, ferric oxides dissolve in the presence of reductants, which can include organic compounds.[6] or bacteria[7] Reductive dissolution is integral to geochemical phenomena such as the iron cycle.[8]
Reductive dissolution does not necessarily occur at the site where the reductant adsorbs. Instead, the added electron travel through the particle, causing reductive dissolution elsewhere on the particle.[9][10]
Nomenclature and formulas
Sometimes, metal-oxygen ratios are used to name oxides. Thus, NbO would be called niobium monoxide and TiO2 is titanium dioxide. This naming follows the Greek numerical prefixes. In the older literature and continuing in industry, oxides are named by adding the suffix -a to the element's name. Hence alumina, magnesia and chromia, are, respectively, Al2O3, MgO and Cr2O3.
Special types of oxides are peroxide, O22−, and superoxide, O2−. In such species, oxygen is assigned higher oxidation states than oxide.
The chemical formulas of the oxides of the chemical elements in their highest oxidation state are predictable and are derived from the number of valence electrons for that element. Even the chemical formula of O4, tetraoxygen, is predictable as a group 16 element. One exception is copper, for which the highest oxidation state oxide is copper(II) oxide and not copper(I) oxide. Another exception is fluoride, which does not exist as one might expect—as F2O7—but as OF2.[11]
Since fluorine is more electronegative than oxygen, oxygen difluoride (OF2) does not represent an oxide of fluorine, but instead represents a fluoride of oxygen.
Examples of oxides
The following table gives examples of commonly encountered oxides. Only a few representatives are given, as the number of polyatomic ions encountered in practice is very large.
Name | Formula | Found/Usage |
---|---|---|
Water (hydrogen oxide) | H 2O |
Common solvent, required by carbon-based life |
Nitrous oxide | N 2O |
Laughing gas, anesthetic (used in a combination with diatomic oxygen (O2) to make nitrous oxide and oxygen anesthesia), produced by nitrogen-fixing bacteria, nitrous, oxidizing agent in rocketry, aerosol propellant, recreational drug, greenhouse gas. Other nitrogen oxides such as NO 2 (nitrogen dioxide), NO (nitrogen oxide), N 2O 3 (dinitrogen trioxide) and N 2O 4 (dinitrogen tetroxide) exist, particularly in areas with notable air pollution. They are also strong oxidisers, can add nitric acid to acid rain, and are harmful to health. |
Silicon dioxide | SiO 2 |
Sand, quartz |
Iron(II,III) oxide | Fe 3O 4 |
Iron ore, rust, along with iron(III) oxide (Fe 2O 3) |
Aluminium oxide | Al 2O 3 |
Aluminium ore, alumina, corundum, ruby (corundum with impurities of chromium). |
Zinc oxide | ZnO | Required for vulcanization of rubber, additive to concrete, sunscreen, skin care lotions, antibacterial and antifungal properties, food additive, white pigment. |
Carbon dioxide | CO 2 |
Constituent of the atmosphere of Earth, the most abundant and important greenhouse gas, used by plants in photosynthesis to make sugars, product of biological processes such as respiration and chemical reactions such as combustion and chemical decomposition of carbonates. CO or Carbon monoxide exists as a product of incomplete combustion and is a highly toxic gas. |
Calcium oxide | CaO | Quicklime (used in construction to make mortar and concrete), used in self-heating cans due to exothermic reaction with water to produce calcium hydroxide, possible ingredient in Greek fire and produces limelight when heated over 2,400 °Celsius. |
See also
![]() |
Look up oxide in Wiktionary, the free dictionary. |
- Other oxygen ions ozonide, O3−, superoxide, O2−, peroxide, O22− and dioxygenyl, O2+.
- Suboxide
- Oxohalide
- Oxyanion
- Complex oxide
- See Category:Oxides for a list of oxides.
- Salt
References
- Foundations of College Chemistry, 12th Edition
- Greenwood, N. N.; & Earnshaw, A. (1997). Chemistry of the Elements (2nd Edn.), Oxford:Butterworth-Heinemann. ISBN 0-7506-3365-4.
- P.A. Cox (2010). Transition Metal Oxides. An Introduction to Their Electronic Structure and Properties. Oxford University Press. ISBN 9780199588947.CS1 maint: uses authors parameter (link)
- http://chemister.ru/Database/properties-en.php?dbid=1&id=4098
- Cornell, R. M.; Schwertmann, U. (2003). The Iron Oxides: Structure, Properties, Reactions, Occurrences and Uses, Second Edition. p. 306. doi:10.1002/3527602097. ISBN 9783527302741.
- Sulzberger, Barbara; Suter, Daniel; Siffert, Christophe; Banwart, Steven; Stumm, Werner (1989). "Dissolution of fe(III)(hydr)oxides in natural waters; laboratory assessment on the kinetics controlled by surface coordination". Marine Chemistry. 28 (1–3): 127–144. doi:10.1016/0304-4203(89)90191-6. ISSN 0304-4203.
- Roden, Eric E. (2008). "Microbiological Controls on Geochemical Kinetics 1: Fundamentals and Case Study on Microbial Fe(III) Oxide Reduction". Kinetics of Water-Rock Interaction. pp. 335–415. doi:10.1007/978-0-387-73563-4_8. ISBN 978-0-387-73562-7.
- Cornell, R. M.; Schwertmann, U. (2003). The Iron Oxides: Structure, Properties, Reactions, Occurrences and Uses, Second Edition. p. 323. doi:10.1002/3527602097. ISBN 9783527302741.
- Yanina, S. V.; Rosso, K. M. (2008). "Linked Reactivity at Mineral-Water Interfaces Through Bulk Crystal Conduction". Science. 320 (5873): 218–222. Bibcode:2008Sci...320..218Y. doi:10.1126/science.1154833. ISSN 0036-8075. PMID 18323417. S2CID 8289796.
- Chatman, S.; Zarzycki, P.; Rosso, K. M. (2015). "Spontaneous Water Oxidation at Hematite (α-Fe2O3) Crystal Faces" (PDF). ACS Applied Materials & Interfaces. 7 (3): 1550–1559. doi:10.1021/am5067783. ISSN 1944-8244.
- Schultz, Emeric (2005). "Fully Exploiting the Potential of the Periodic Table through Pattern Recognition". J. Chem. Educ. 82 (11): 1649. Bibcode:2005JChEd..82.1649S. doi:10.1021/ed082p1649.