Garudimimus
Garudimimus (meaning "Garuda mimic") is a genus of ornithomimosaur that lived in Asia during the Late Cretaceous. The genus is known from a single specimen found in 1981 by a Soviet-Mongolian paleontological expedition in the Bayan Shireh Formation and formally described in the same year by Rinchen Barsbold; the only species is Garudimimus brevipes. Several interpretations about the anatomical traits of Garudimimus were made in posterior examinations of the specimen, but most of them were criticized during its comprehensive redescription in 2005. Extensive undescribed ornithomimosaur remains at the type locality of Garudimimus may represent additional specimens of the genus.
Garudimimus | |
---|---|
![]() | |
Mounted holotype in the Mongolian Natural History Museum (note incorrect number of caudal vertebrae; only four are reported in the holotype) | |
Scientific classification ![]() | |
Kingdom: | Animalia |
Phylum: | Chordata |
Clade: | Dinosauria |
Clade: | Saurischia |
Clade: | Theropoda |
Clade: | Maniraptoriformes |
Clade: | †Ornithomimosauria |
Genus: | †Garudimimus Barsbold, 1981 |
Type species | |
†Garudimimus brevipes Barsbold, 1981 |
The only known specimen of Garudimimus was a medium-sized animal measuring nearly 3.5 m (11 ft) in length and weighing about 98 kg (216 lb). It was an ornithomimosaur with a mix of basal and derived features; unlike primitive ornithomimosaurs, both upper and lower jaws were toothless, a trait that is often reported in more derived ornithomimids. Garudimimus had relatively short and stocky hindlimbs, robust feet, and a reduced ilia. The foot had four toes with the first one very reduced, whereas ornithomimids were three-toed with the first toe lost. The toothless skull has very straight jaws ending in a more rounded snout tip than that of other genera. It was previously thought that this primitive ornithomimosaurian possessed a lacrimal "horn" at the top of the skull, in front of the eye socket. However, the redescription of the only specimen has shown that this structure was simply the distorted left prefrontal bone. Another early interpretation was the metatarsus reconstructed with an arctometatarsalian condition.
With the first description in 1981, Garudimimus was identified as a primitive ornithomimosaurian within its own family. However, with the description of new specimens of Deinocheirus in 2014, it was found that the latter was the sister taxon of Garudimimus, grouping within the Deinocheiridae—ornithomimosaurs not adapted for running or agile movements. But this placement has also been found to be unlikely. The pelvic girdle and hindlimbs of Garudimimus show that the musculature of the legs was not as well-developed as in the fast-running ornithomimids, therefore, indicating poor cursorial capacities. Like other members of Ornithomimosauria, Garudimimus was an omnivore/herbivore with a reduced bite force that was compensated by a horny beak.
History of discovery
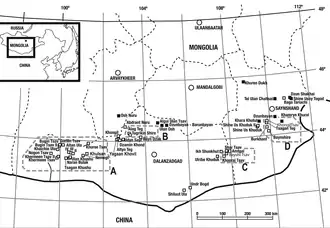
In 1981, during a Soviet-Mongolian paleontological expedition to the Gobi Desert, a relatively small theropod skeleton was discovered by the team at Bayshi Tsav in the Late Cretaceous Bayan Shireh Formation, Southeastern Mongolia. The remains are catalogued as MPC-D 100/13 (Mongolian Palaeontological Center; originally GIN 100/13) and represent a rather complete and articulated skeleton. In the same year this specimen was formally and briefly described by the Mongolian paleontologist Rinchen Barsbold as the holotype of the genus and species Garudimimus brevipes. The generic name, Garudimimus, combines a reference to Garuda which are legendary winged creatures from Mongolian Buddhist mythology and Latin mimus (meaning mimic). The specific name is derived from Latin brevis (meaning short) and pes (meaning foot), referring to the short metatarsus. Barsbold identified Garudimimus as an ornithomimosaurian taxon but noted it was more primitive than ornithomimids and gave it its own family, the Garudimimidae.[1] Barsbold described additional remains of the holotype specimen in 1983, and later in 1990 with Halszka Osmólska.[2][3]
The holotype consist of the skull in its entirety, 8 cervical vertebrae (including the atlas and axis), 9 dorsal vertebrae, 6 sacral vertebrae and 4 caudal vertebrae, some ribs, both ilia, pubes, femora, tibiae, fibulae, and a virtually complete left pes of a sub-adult individual.[1][4]
In 1988 the North American author Gregory S. Paul illustrated Garudimimus with a prominent nasal horn unlike any other ornithomimosaur, considering this feature as actually preserved.[5] Another interpretation was made also in 1988 by Philip J. Currie and Dale Alan Russell who referred the metatarsus of the holotype to Oviraptor sp. and reconstructed it with an arctometatarsal—a condition where the upper end of the third metatarsal is narrowed between the surrounding metatarsals—structure.[6] In 1992 Thomas R. Holtz followed this interpretation and suggested the metatarsus could have been arctometatarsalian and was just disarticulated as preserved.[7] Supporting an arctometatarsalian condition, Currie and David A. Eberth in 1993 claimed that part of the Archaeornithomimus (an ornithomimosaur from the nearby Iren Dabasu Formation) material belonged to Garudimimus based on the assumed arctometatarsalian condition, presence of the vestigial digit I and the proportions of metatarsals II, III, and IV. They pointed out that the metatarsals are crushed and metatarsal III is set back from the extensor surface of metatarsus.[8] In 1994 Holtz suggested some similarities between the metatarsus of Garudimimus and Chirostenotes.[9]
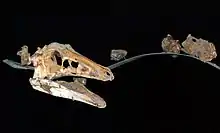
In 1994, Bernardino P. Pérez-Moreno with colleagues described the primitive ornithomimosaur Pelecanimimus and identified the presence of a crest in the holotype specimen. They claimed a similar trait in Garudimimus which was represented by a nasal horn.[10] In a comprehensive redescription in 2005 at the hands of Yoshitsugu Kobayashi and Barsbold, numerous of the previous statements were refuted. The supposed orbital horn is actually the disarticulated left prefrontal bone and verified that the metatarsus did not suffer taphonomical (changes during decay and fossilisation) distortion and is non-arctometatarsalian. Moreover, Kobayashi previously showed that in addition to actually lacking an arctometatarsalian condition, the metatarsal ratios are different to Archaeornithomimus.[4] Although Garudimimus is solely known from MPC-D 100/13, additional specimens may potentially be present among a large bonebed composed of at least five individuals in Bayshi Tsav and several undescribed ornithomimosaurs from other localities in the Bayan Shireh Formation.[11][12][13]
Description
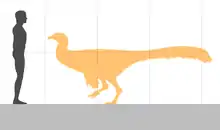
Garudimimus (based on the holotype specimen) is estimated to have been around 3.5 m (11 ft) weighing approximately 98 kg (216 lb).[14][15] Several unfused vertebrae indicates that the holotype was likely a still-growing sub-adult animal.[4] Some of the distinguishing traits of this taxon include the jaw articulation positioned more backwards than the postorbital bar (arched bone around the orbit, also referred as the postorbital), depressions on the upper surface of the supraoccipital at the back of the skull, paired depressions on the lateral surfaces of the neural spines of the anterior caudal vertebrae, and a deep groove at the upper end of lateral surface of pedal phalanges III-1 and III-2 (first and second phalanxes of the third digit of the foot).[4]
Based on feather impressions of several specimens of Ornithomimus, Garudimimus likely developed a shaggy feathering mostly similiar to that of large paleognath birds such as ratites.[16][17]
Skull
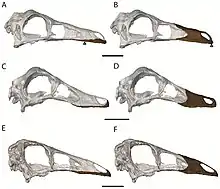
The holotype skull is relatively well-preserved and though the left side is more damaged than the right, the former preserves delicate sclerotic rings (bony plates that support the eyeball). The jaws were edentulous (toothless), with both premaxilla and dentary bones lacking alveoli (teeth sockets).[4] From a dorsal perspective, the skull is greatly crushed from side to side, measuring 3.6 cm (36 mm) wide. When retrodeformed, the skull gets notably expanded with a width of 4.6 cm (46 mm).[18] In the original crushed skull, the infratemporal fenestra has an irregular shape due to the displacement of some elements. The upper curvature of this fenestra is poorly developed. The edges of the premaxilla are thin and sharply developed. On the lateral sides of this bone numerous foramina (small holes) can be found. The premaxilla borders most of the external narial opening but not the posterior edge. When seen from a lateral view, the bottom border of the premaxilla is straight and the contact with the maxilla lies backwards to the posterior end of the nostrils. The lateral premaxilla-maxilla suture forms a right angle to the bottom border of the upper jaw. The dorsal extension of the maxillary process (bony extension) ends posterior to the anterior border of the antorbital fossa. The palatal shelf of both premaxillae has an incisive foramen and a pair of foramina. The lateral surface of the main body of the maxilla is rough as in other ornithomimosaurs. Both promaxillary and maxillary fenestrae are poorly developed. The upper extension of the maxilla joins with the lacrimal bone at the center of the antorbital fossa. In lateral view, the bottom border of the maxilla is also straight. As in derived ornithomimids, the bottom surface has numerous foramina.[4] The edentulous condition of both premaxilla and dentary with the addition of numerous foramina in their surfaces indicate that a prominent rhamphotheca (beak) was present in life.[18] The nasal bone is elongated and gives form to the posterior border of the external narial opening. It narrows towards the frontal contact, and its posterior end lies between the posterior ends of the prefrontal and lacrimal. Some foramina are present on the top surface.[4]

The lacrimal bone is semi-T-shaped and the anterior tip is damaged with this extension being spaced by the nasolacrimal canal. The surface that is situated on the lateral side and towards the posterior end of the bottom extension has a posterior opening for the nasolacrimal canal—also reported in "Gallimimus mongoliensis". On the inner surface of the lacrimal, a large triangular-shaped depression can be found. The posterior process of the lacrimal narrows and inserts into a depression on the prefrontal. The prefrontal has an anterior process that unites to the lower surface of the main body of the lacrimal. The open dorsal area of the prefrontal is slightly reduced than that of the lacrimal. The anterior process is divided in two, and the inner branch is much larger than the lateral one. In a posterior perspective, the orbital rim of the prefrontal thickens to the bottom and lacks foramina.[4] As preserved, the orbital is a large cavity with a height of 5.95 cm (59.5 mm). When retrodeformed, it gets slightly larger being 6.1 cm (61 mm) wide.[18] Though the right side is more complete, the left side of the skull preserves at least 11 articulated scleral plates forming the sclerotic ring. The radius of the ring is approximately 2.86 cm (28.6 mm) inside and 4.12 cm (41.2 mm) outside. The breadth of each plate is about 0.8 cm (8.0 mm). The anterior tip of the jugal is not preserved at all, and the sutures for the lacrimal and maxilla are not well-developed. The jugal lengthens to the end and in lateral view, the jugal is very flat and has a rather short posterior process. The lateral surface has a shallow depression to connect with the anterior process of the quadratojugal, however, it is located before the posterior edge of the orbit.[4]
The quadratojugal is a large and triangular-shaped bone—contrasting with the L-shaped quadratojugal of most ornithomimosaurs. Although the tip of the dorsal process is damaged, it was apparently longer than the anterior one.[4] On the posterior surface, the quadrate preserves a large depression that is positioned at its mid-height. This depression is oval in shape, measuring 1.2 cm (12 mm) long and 0.4 cm (4.0 mm) wide. A pneumatic foramen is also present in the bottom area of the depression.[19] Near the bottom region to the depression, the lateral border of the quadrate has a tract for the paraquadrate foramen. Both mandibular condyles are equal in size and well-separated by a groove. The pterygoid extension is triangular-shaped in lateral view and large, measuring at least 2.9 cm (29 mm) long at the level of the pneumatic foramen. In a top view, the frontals are triangular and the anterior flattened region of the frontals is inclined towards an anterior direction. To the posterior end, the frontals form a single dome-like structure. On the inner sides, the parietals are flattened in the posterior portion of the skull roof. The skull roof is more wider than long though. The posterior process is stiffened and developed in this direction.[4]
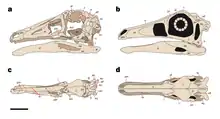
The postorbital is a sharply developed bone of well-distributed thickness in lateral view. The suture with the frontal originates from the upper posterior region of the orbit and the postorbital-parietal suture stretches to the supratemporal fenestra. To the lower direction, the bottom process is narrow. The bottom tips of the squamosal are damaged and the tall anterior process is connected in the inner side to the posterior process of the postorbital. Both anterior and lower extensions of the squamosal are elongated in a lateral view. The edentulous lower jaws are very complete and well-preserved. The dentary is the longest mandibular element measuring 18.3 cm (183 mm) long and is more thickened to the posterior end.[4] Given that Garudimimus had a downturned dentary, the jaws do not occlude across the entire oral border and this would limit the functionality of the jaws. The presence of a rhamphotheca would likely fill the gap to form an occlusal surface.[18] The upper border of the dentary is well-developed with a cutting edge, and is side to side rounded. Numerous foramina can be found on the lateral surfaces of the dentary tip. The symphysis (bone union) is poorly developed, showing that the interdentary articulation was not robust. Both dentaries form a round shovel-like shelf at the symphysis that is wide and deep. On the inner surface a Meckelian groove can be found, but it is covered by the splenial. The dentary has two posterior processes and one at the anterior border of the external mandibular fenestra.[4]
The splenial has its deepest at the posterior end of the cutting edge of the dentary and narrows on the anterior end. Its posterior edge is somewhat concave and inclined forwards from an inner view. The posterior end thins out to the bottom area of the prearticular. A second large mandibular element is represented by the surangular. Its dorsal extension is slightly flattened and extends more to the front than to the anterior edge of the mandibular fenestra. The dorsal edge of the surangular gives form to an inner projection along its entire body. This edge has a ridge-like structure to joint with the mandibular condyle of the quadrate. The surangular lacks any traces of a posterior surangular foramen. It only participates in anterior half of the lateral surface of the retroarticular process. The retroarticular process (a backwards bony extension) points gently upward at its posterior tip. Compared to the surangular, the angular is smaller and borders the external mandibular fenestra towards the bottom. The straight suture between these bones extends from the posterior end of the external mandibular fenestra to the posterior end of the mandible. The anterior process of the angular is elongated and meets the dentary in this direction. The prearticular is large and stocky. The anterior end of this bone lies posterior to the anterior edge of the external mandibular fenestra and cuts towards the rear. The articular surfaces for the mandibular condyles of the quadrate are well separated by a ridge-like structure. The top regions of this ridge are located in the front and towards the center, and situated on the lateral side and toward the end. A large and deep pneumatopore can be found on the inner surface.[4]
Postcranial skeleton
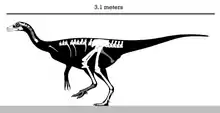
Most cervical vertebrae have eroded surfaces and are side to side crushed; the most complete cervical is represented by the axis. Aside from the atlas and axis, six more vertebrae are preserved and can be attributed to third through sixth cervicals, but the last two are of unknown position. The partial intercentrum of the atlas is articulated near the lower region of the axis and the left half of the neural arch is also present. The central length of the axis is rather short, measuring approximately 3 cm (30 mm) long and 1.8 cm (18 mm) high—probably a primitive condition in ornithomimosaurs. Its posterior articular surface is concave and taller than wide. On the lateral surfaces of the centrum, a pneumatic fossa is absent. The neural spine is tall and very rounded having a ligament scar on the posterior border, and the axial neural arch has a pneumatic fossa at the mid-length of its centrum. Cervicals third to fifth are articulated but only preserving the right side of the neural arches and cervicals fourth with sixth are the most complete. The neural spines are narrow and located towards the end. The two last cervicals are articulated and their exact positions are unclear, however, their elongated postzygapophyses suggest that they belong to the posterior series. Nevertheless, the anterior cervical of these two is probably the seventh cervical. Nine consecutive dorsal vertebrae are preserved and based on Ornithomimus, they probably represent fourth through twelfth dorsals. They progressively increase in size and some sutures around the dorsals are not completely fused, suggesting that the holotype specimen is not fully mature. Along with the size, the neural spines become progressively taller in more posterior dorsals, but their lengths are nearly equal. In a lateral view, all spines are slightly inclined towards the end except the twelfth, which is forwards fractured. The dorsal centra are larger in more posterior vertebrae with amphicoelous (strongly concave) facets and the lateral surfaces lack pneumatization. On the bottom surfaces in the fourth and fifth dorsal centra, large ridge-like structures can be found. In the lateral surfaces of the centra, both anterior and posterior edges have ligament scars.[4]
The sacrum is composed of six sacral vertebrae of which the first and sixth have sacral ribs attached to the inner surface of the ilium. The total length of the sacrum—31 cm (310 mm)—is nearly equal to that of the ilium. Most sacral centra are roughly equal in length except for the fourth one. There are some fused sections of the sacrum, such as the first and second, and the third and fourth sacrals, but some areas are partially unfused. The neural spines of the first to fifth sacrals are fused and the neural spine of the sixth is missing. This irregular ossification may indicate a sub-adult individual. The sacral vertebrae lack pneumatization and in a lateral view ligament scars can be found on the anterior borders of the first and sixth sacral vertebrae, and posterior edges of the fifth and sixth sacral vertebrae. The first four caudal vertebrae are preserved with all the sutures between the centra and neural arch open. The neural spines become progressively shorter and have straight and horizontal dorsal borders with the anterior and posterior borders grooved. The lengths of the centra are equal and on their bottom surfaces, the caudal vertebrae have paired projections on either side of the sulci (fissures) for the chevron articulation.[4] Putative large foramen on the right side of the neural arch of the anterior caudal vertebrae and deep fossae situated on both the right back and the side surface of an anterior neural arch could indicate caudal pneumaticity in Garudimimus.[20]
The third and fourth cervical chevrons are partially preserved only with their posterior and anterior parts, respectively. Nine dorsal ribs are present in the holotype and have a short tuberculum and long capitulum. Fifteen gastralia fragments are preserved but their exact positions are unknown. These are segmented into, at least, lateral and inner parts. Most sacral ribs are poorly developed. The pelvic girdle is nicely preserved, however, both ischia are not present. Both ilia are gently crushed and the dorsal edges of the ilia lie close to each other with the anterior end being hook-shaped. In a lateral view, the ischiadic peduncle (extension behind the acetabulum to attach the ischium) is wedge-shaped and pointed, and wide in from the bottom view as in most other ornithomimosaurs. The brevis fossa (caudofemoralis brevis muscle attachment) is large but very shallow, in addition, the ilium is not as extensive as in ornithomimids. The cuppedicus fossa (concavity between the preacetabular blade and pubic peduncle) is smaller than the brevis fossa. The pubis is a large element measuring 39 cm (390 mm) in length. In lateral view, the upper end has an elongated hook-like structure on the bottom border of the pubis–ischium contact. Its shaft is nearly straight and the posterior extension of the pubic boot (large projection at the end) is longer than the anterior extension. Both pubes are fused.[4]

The femur measures 37.1 cm (371 mm) and from a lower view, the total length of the femur head is more than half of the width lying across of the its upper end. On the anterior border of the lesser trochanter, a large additional trochanter can be found and these trochanters are divided by a sulcus. The fourth trochanter is positioned at roughly 1/3 of the femur length from the upper end. The tibial articular protuberance of the lateral condyle is developed on a posterior direction. The tibiotarsus-femur length ratio is 1.1 and its upper end has equally developed lateral and inner posterior condyles. The fibula is slightly shorter than the tibia (36 cm (360 mm) and 38.8 cm (388 mm) respectively). This bone is more flattened than other limb elements and the shaft is very thin. Both astragalus and calcaneum are attached in the left tibia, but the calcaneum appears to be slightly damaged. Among the lower tarsal elements preserved are III and IV. The tarsal III articulates to the upper surface of the third metatarsal and partially with the second metatarsal. Tarsal IV covers most of the upper surface of the fourth metatarsal.[4]
In the pes formula, Garudimimus strongly differs from the ornithomimid condition by having five metatarsals. Metatarsal I is the first and smallest element of the metatarsus with a more flattened and pinched shape. The articular surface between metatarsal I and metatarsal II is located on the posterior surface of the latter. Metatarsals II and IV are subequal in length and metatarsal III is the longest (22.9 cm (229 mm)). The lower end of metatarsal III is wider in a frontal view and is not pinched at its upper end, therefore indicating a non-arctometatarsalian condition.[4] This trait is partially shared with the large Beishanlong and Deinocheirus.[21] Metatarsal V is a very reduced and thin element that has no functional significance and articulates with the posterior surface of metatarsal IV. Only eight pedal phalanges and two left pedal unguals are present with the holotype. The feet had four primitive toes with the first being the smallest. Most phalanges are very similar in shape being expanded in their anterior and posterior ends with rounded lateral condyles and concave anterior articular surfaces. They become progressively shorter from the second to the fourth digit.[4] Both preserved unguals have a recurved shape, which differs from the straight shape in ornithomimids.[21] The ungual from the fourth digit has a prominent flexor tubercle (flexor tendons attachment). The second digit ungual is similar to the former in having a strong flexor tubercle but has a more irregular shape.[4]
Classification
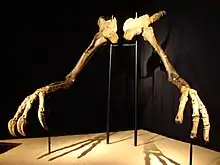
In 1981, Barsbold assigned Garudimimus to a separate Garudimimidae given the unusual traits in the taxon. However, he identified these as clearly primitive.[1] With the redescription of the holotype in 2005, more diagnostic characters for Garudimimus were provided. Kobayashi and Barsbold noted that this taxon shared traits with primitive and derived ornithomimosaurs such as four-toed feet and toothless jaws. Their phylogenetic analysis recovered Ornithomimidae as the sister taxon of Garudimimus.[4] In 2014, new and fairly complete specimens of the long-enigmatic Deinocheirus were described by Yuong-Nam Lee and colleagues. The remains showed that Deinocheirus was in fact an ornithomimosaur taxon—but aberrantly different—and also allowed to define the older Deinocheiridae as the family including all taxa sharing a more recent common ancestor with Deinocheirus than with Ornithomimus. Lee and team performed a large phylogenetic analysis for the Deinocheiridae and the three members were found in this grouping: Beishanlong, Garudimimus and Deinocheirus. Their relationships were mainly represented by various anatomical features in the limbs, but the latter two were strongly related as they both have skull and body remains. The resulting cladogram suggested that ornithomimosaurian dinosaurs diverged into two major lineages in the Early Cretaceous: Deinocheiridae and Ornithomimidae. Unlike the fast-running ornithomimids, deinocheirids were not built for running and the anatomical traits of Deinocheirus when compared to other ornithomimosaurs can be explained by the notable large sizes achieved in this taxon.[21] More recently, in 2019 Hartman and colleagues described the new paravian Hesperornithoides performing an extensive phylogenetic analysis for the Coelurosauria. In the case of ornithomimosaurs, the Garudimimidae was recovered as a family containing Garudimimus and close relatives. Garudimimus was recovered in a rather derived position as the sister taxon of Beishanlong. This analysis found close relationship between Deinocheirus and Garudimimus to be unlikely, with the former recovered at the near base of Ornithomimosauria.[22]
Left cladogram per Lee and colleagues 2014,[21] right cladogram per Hartman and colleagues 2019:[22]
|
|
In 2020, Serrano-Brañas and team described and named the new deinocheirid genus Paraxenisaurus from the Cerro del Pueblo Formation. This is the first member of the group to be found outside Asia and its discovery could indicate that deinocheirids originated in Laurasia (northern supercontinent during the Mesozoic) or that they dispersed across polar regions in the Northern Hemisphere; a similar interchange is also known to have occurred in other dinosaur groups with Asian affinities during the Campanian-Maastrichtian ages. The team found Harpymimus to be a rather primitive deinocheirid, however, Beishanlong was placed outside the group. Garudimimus and Deinocheirus were recovered again as closely related taxa with the addition of Paraxenisaurus in a polytomy.[23]
Paleobiology
Daily activity
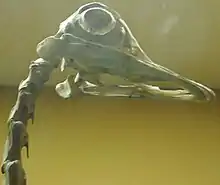
Limb proportions, pedal unguals morphology and the extension of the ilium of Garudimimus suggest that it was not adapted for speed as in the more cursorial ornithomimids, which are characterized by powerful thighs and straight unguals.[4][21] In 2011, Lars Schmitz and Ryosuke Motani measured the dimensions of the sclerotic ring and eye socket in fossils specimens of dinosaurs and pterosaurs, as well as some living species. They noted that whereas diurnal (photopic) animals have smaller sclerotic rings, nocturnal (scotopic) animals tend to have more enlarged rings. Cathemeral (mesopic) animals—which are irregularly active throughout the day and night—are between these two ranges. Schmitz and Motani separated ecological and phylogenetic factors and by examining 164 living species, they noticed that eye measurements are quite accurate when inferring diurnality, cathemerality or nocturnality in tetrapods. The results indicated that Garudimimus was a cathemeral herbivore with other predatory theropods being mainly nocturnal. Large herbivorous dinosaurs were found to have been cathemeral most likely because of the extended amounts of time during foraging and thermoregulation factors. Birds and pterosaurs were mostly diurnal with some nocturnal exceptions. They concluded that the ecological niche was a main driver in the development of diel activity.[24]
However, this analysis was criticized in the same year by Hall and colleagues arguing against the methods employed by Schmitz and Motani in that there is a considerable degree of similarities on scleral anatomy between diurnal, cathemeral and nocturnal animals. Moreover, soft tissues like the cornea are essential in figuring out how much light can enter the eye, which is clearly absent in fossil specimens.[25] Schmitz and Motani made a response to this comment affirming their research and methodology properly categorized extinct dinosaur behaviors based on extant species. They cited the exterior border of the scleral ring as a reliable source when reconstructing visual capabilities.[26]
Senses
In 2019, Graham M. Hughes and John A. Finarelli analyzed the olfactory bulb ratio in modern birds and preserved skulls of several extinct dinosaur species to predict how many genes would have been involved in the olfactory strength of these extinct species. Their analysis found that most ornithomimosaurs had relatively weak senses of smell; Garudimimus had roughly 417 genes encoding its olfactory receptors and an olfactory bulb ratio of 28.8, indicating a poorly developed sense of smell. Given that these values were more pronounced in larger dinosaur species, such as tyrannosaurids, Hughes and Finarelli pointed out that as dinosaurian lineages became larger, the size of the olfactory bulb also increased, which may suggest olfaction as the dominant sensory modality in non-avian dinosaurs.[27]
Feeding habits

Kobayashi and Barsbold stated in 2005 that the absence of dentition on the lower jaw and the recurved shape of the same, indicates that Garudimimus was fairly more similar to ornithomimids when consuming food than to other primitive ornithomimosaurs. Like ornithomimids, the anterior parts of the upper and lower jaws of Garudimimus were both covered by a beak and may have been used for plucking food. In addition, the sharp edges on the middle portions of the jaws helped for cutting food.[4] Moreover, Garudimimus and kin are widely regarded as herbivore or at least omnivore dinosaurs by their peculiar anatomical traits—including the presence of a beak.[28][24]
In 2015, Andrew R. Cuff and Emily J. Rayfield performed CT scans on taphonomically distorted specimen skulls of Garudimimus, Ornithomimus and Struthiomimus in order to be retrodeformed—a method used to produce the original state of a deformed object. The scans and relatively completeness of the specimens allowed the reconstruction of the adductor (motion-inducing) musculature of the lower jaw, which was then mapped onto the skulls. Additionally, the extent of the rhamphotheca (beak) was reconstructed into small—based on a ratite bird, the ostrich—and large morphs. Among the three genera studied, Garudimimus had the most reduced bite force, found to produce 19 N at the tip of the beak and 23.9 N at the mid-length of the same. However, this taxon had the most modified muscle mechanical advantage and moment arm—a measure of the effectiveness of a muscle at contributing to a particular motion over a range of configurations. With this consideration, the most significant muscular difference between Garudimimus and the two ornithomimids was found in that most muscles are more mechanically advantageous in the latter two; this is likely caused due to the longer skull in Garudimimus. Cuff and Rayfield pointed out that the bite force of Garudimimus may be limited for having to use the mandible of Struthiomimus in the reconstructions or that MPC-D 100/13 is a sub-adult individual. Tentatively, they agreed in that relatively low bite forces combined with a rhamphotheca could be used to hold plant material during feeding in ornithomimosaurs, all of this whilst neck musculature provided enough force to pluck away vegetation.[18]
In 2019, David J. Button and Lindsay E. Zanno performed a large phylogenetic analysis based on skull biomechanical characters—provided by 160 Mesozoic dinosaur species—to analyze the multiple emergences of herbivory among non-avian dinosaurs. Their results found that herbivorous dinosaurs mainly followed two distinct modes of feeding, either processing food in the gut—characterized by relatively gracile skulls and low bite forces—or the mouth, which was characterized by features associated with extensive processing such as high bite forces and robust jaw musculature. Garudimimus and Deinocheirus, along with caenagnathid, diplodocoid, ornithomimid, therizinosaurid and titanosaur dinosaurs, were found to be in the former category, indicating that Garudimimus had indeed low bite forces and relied mostly on its stomach to process food. Large body sizes were achieved independently in deinocheirids and ornithomimids, and larger sizes offer advantages to herbivore animals, such as an increased resistance to fasting and more ingestion. Hence, these habits may indicate a more herbivorous life-style in deinocheirids and ornithomimids. However, Button and Zanno pointed out the relationship between herbivory and size is not always related and there is not a lineal trend of increasing mass in ornithomimosaurs. Furthermore, specific niches were poorly exploited for most ornithomimosaurian dinosaurs with only Deinocheirus showing an opportunistic omnivory. Button and Zanno concluded that feeding strategies convergently evolved in unrelated herbivorous dinosaurs over the time.[29]
During the same year, Ali Nabavizadeh studied the jaw musculature in herbivorous dinosaurs and concluded that some were primarily orthal feeders—moving their jaws up and down—including theropods, sauropodomorphs, primitive ornithischians, and some stegosaurs. Nabavizadeh noted that ornithomimosaurs were orthal feeders and raised their jaws isognathously whereby the upper and lower teeth of each side occluded (contacted each other) at once. Deinocheirus and Garudimimus have unique attachment sites for the muscles that open and close the jaws, which are composed by a downwards-expanded, triangular adductor musculature complex attaching to a forwards expanding surangular bone. This, working together with a flared jugal flange, allows more cavity for the adductor muscle. With such elongate snout, the bite forces of both deinocheirids were low with reduced mechanical advantage, however, if this muscle extended farther forwards then this would have added strength to the temporal muscle.[30]
Paleoenvironment
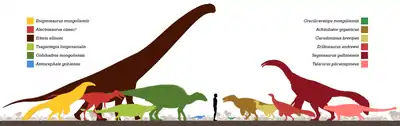
The known remains of Garudimimus were unearthed from the Late Cretaceous Bayan Shireh Formation at the Bayshi Tsav locality.[1] This formation is mainly composed by claystones and sandstones with concretions and characterized by mudstones and yellowish-brown medium grained sandstones. The presence of caliche, fluvial and lacustrine sedimentation at various localities indicates that this formation was deposited by meandering rivers and other large water bodies on an alluvial plain (flat land consisting of sediments deposited by highland rivers) under a semi-arid climate.[31][32] Bayshi Tsav itself, is interpreted to have been deposited under fluvial environments.[33] Using calcite U–Pb measurements, the age of the Bayan Shireh Formation has been dated from about 95.9 ± 6.0 million to 89.6 ± 4.0 million years ago during the Cenomanian-Santonian stages.[34]
Numerous scale-like cross-stratifications at the Baynshire locality are indicators of large meanders, and the vast majority of these water bodies may have drained the eastern part of the Gobi Desert.[32] These humid environments are further supported by the discovery of aquatic reptiles like turtles and crocodylomorphs, and ostracods.[35][36] Based on the similar fossil assemblages, a correlation between the Bayan Shireh and Iren Dabasu formations has been proposed by numerous authors.[31][8][37] Furthermore, the Iren Dabasu Formation has been dated about 95.8 ± 6.2 million years ago.[38]

Garudimimus shared its habitat with multiple dinosaurian fauna, such as the herbivorous ornithischians Gobihadros, Graciliceratops and Talarurus.[39][40] Other theropods are represented by the predator of medium-sized prey Achillobator,[41] and the therizinosaurids Erlikosaurus and Segnosaurus.[42] Erketu may have been the tallest herbivore within the fauna.[43] Several species were separated by niche differentiation, such as Erlikosaurus and the large Segnosaurus,[42] or the grazer Talarurus and browser Tsagantegia, another ankylosaurid from the formation.[40] In terms of biodiversity, therizinosaurs were some of the most abundant theropods with multiple described and undescribed specimens across the formation.[36] However, the high concentration of scattered hadrosauroid remains—particularly at Baynshire—appear to reflect a great abundancy;[33] Gobihadros is known from at least three different localities.[44] Though Garudimimus is currently the only ornithomimosaur taxon named, the Bayan Shireh Formation has produced locally abundant ornithomimosaurs at Bayshi Tsav.[45][46][11] Among them, the informal "Gallimimus mongoliensis" is one of the most complete, however, this species does not belong to the much younger Gallimimus and represents an entire new taxon.[47]
See also
References
- Barsbold, R. (1981). "Беззубые хищные динозавры Монголии" [Toothless carnivorous dinosaurs of Mongolia]. Transactions, Joint Soviet–Mongolian Palaeontological Expedition (in Russian). 15: 28−39. S2CID 195060070. Translated paper
- Barsbold, R. (1983). "Хищные динозавры мела Монголии" [Carnivorous dinosaurs from the Cretaceous of Mongolia] (PDF). Transactions of the Joint Soviet-Mongolian Paleontological Expedition (in Russian). 19: 1−120. Translated paper
- Barsbold, R.; Osmólska, H. (1990). "Ornithomimosauria". In Weishampel, D. B.; Osmolska, H.; Dodson, P. (eds.). The Dinosauria (1st ed.). Berkeley: University of California Press. pp. 225−244. ISBN 9780520067271.
- Kobayashi, Y.; Barsbold, R. (2005). "Reexamination of a primitive ornithomimosaur, Garudimimus brevipes Barsbold, 1981 (Dinosauria: Theropoda), from the Late Cretaceous of Mongolia" (PDF). Canadian Journal of Earth Sciences. 42 (9): 1501−1521. Bibcode:2005CaJES..42.1501K. doi:10.1139/e05-044. hdl:2115/14579.
- Paul, G. S. (1988). "The Enigmatic Ostrich-Mimics". Predatory Dinosaurs of the World. Simon & Schuster. p. 383. ISBN 978-0-671-61946-6.
- Currie, P. J.; Russell, D. A. (1988). "Osteology and relationships of Chirostenotes pergracilis (Saurischia, Theropoda) from the Judith River (Oldman) Formation of Alberta, Canada". Canadian Journal of Earth Sciences. 25 (7): 272−286. Bibcode:1988CaJES..25..972C. doi:10.1139/e88-097.
- Holtz, T. R. (1992). An unusual structure of the metatarsus of Theropoda (Archosauria: Dinosauria: Saurischia) of the Cretaceous (PhD thesis). Yale University. p. 347.
- Currie, P. J.; Eberth, D. A. (1993). "Palaeontology, sedimentology and palaeoecology of the Iren Dabasu Formation (Upper Cretaceous), Inner Mongolia, People's Republic of China". Cretaceous Research. 14 (2): 127−144. doi:10.1006/cres.1993.1011.
- Holtz, T. R. (1994). "The arctometatarsalian pes, an unusual structure of the metatarsus of Cretaceous Theropoda (Dinosauria: Saurischia)". Journal of Vertebrate Paleontology. 14 (4): 480−519. doi:10.1080/02724634.1995.10011574. JSTOR 4523590.
- Pérez-Moreno, B. P.; Sanz, J. L.; Buscalioni, A. D.; Moratalla, J. J.; Ortega, F.; Rasskin-Gutman, D. (1994). "A unique multitoothed ornithomimosaur dinosaur from the Lower Cretaceous of Spain" (PDF). Nature. 370 (6488): 363−367. Bibcode:1994Natur.370..363P. doi:10.1038/370363a0. S2CID 4302245.
- Chinzorig, T.; Kobayashi, Y.; Saneyoshi, M.; Tsogtbaatar, K.; Batamkhatan, Z.; Ryuji, T. (2017). "Multitaxic bonebed of two new ornithomimids (Theropoda, Ornithomimosauria) from the Upper Cretaceous Bayanshiree Formnation of southeastern Gobi desert, Mongolia". Journal of Vertebrate Paleontology. Program and Abstracts: 97.
- Chinzorig, T.; Kobayashi, Y.; Tsogtbaatar, K.; Currie, P. J.; Takasaki, R.; Tanaka, T.; Iijima, M.; Barsbold, R. (2018). "Ornithomimosaurs from the Nemegt Formation of Mongolia: manus morphological variation and diversity". Palaeogeography, Palaeoclimatology, Palaeoecology. 494: 91–100. Bibcode:2018PPP...494...91C. doi:10.1016/j.palaeo.2017.10.031.
- Tsogtbaatar, K. (2019). Evolution, diversity, and disparity of ornithomimosaurs (Dinosauria: Theropoda) from the Upper Cretaceous of Mongolia (PDF) (PhD thesis). Hokkaido University. hdl:2115/74432.
- Temperton, J.; Rosewarne, G.; Kindersley, D. (1993). Kindersley, D. (ed.). The Visual Dictionary of Dinosaurs. Eyewitness Visual Dictionaries. Pennsylvania State University: DK CHILDREN. pp. 1−64. ISBN 9780751310122. LCCN 92053446. OCLC 38325967.
- Zanno, L. E.; Makovicky, P. J. (2013). "No evidence for directional evolution of body mass in herbivorous theropod dinosaurs". Proceedings of the Royal Society B. 280 (1751): 20122526. doi:10.1098/rspb.2012.2526. PMC 3574415. PMID 23193135.
- Zelenitsky, D. K.; Therrien, F.; Erickson, G. M.; DeBuhr, C. L.; Kobayashi, Y.; Eberth, D. A.; Hadfield, F. (2012). "Feathered Non-Avian Dinosaurs from North America Provide Insight into Wing Origins". Science. 338 (6106): 510–−514. Bibcode:2012Sci...338..510Z. doi:10.1126/science.1225376. PMID 23112330. S2CID 2057698.
- Van der Reest, A. J.; Wolfe, A. P.; Currie, P. J. (2016). "A densely feathered ornithomimid (Dinosauria: Theropoda) from the Upper Cretaceous Dinosaur Park Formation, Alberta, Canada". Cretaceous Research. 58: 108−117. CiteSeerX 10.1.1.710.3240. doi:10.1016/j.cretres.2015.10.004.
- Cuff, A. R.; Rayfield, E. J. (2015). "Retrodeformation and muscular reconstruction of ornithomimosaurian dinosaur crania". PeerJ. 3: e1093. doi:10.7717/peerj.1093. PMC 4512775. PMID 26213655.
- Hendrickx, C.; Araújo, E.; Mateus, O. (2015). "The non-avian theropod quadrate I: standardized terminology with an overview of the anatomy and function". PeerJ. 3: e1245. doi:10.7717/peerj.1245. PMC 4579021. PMID 26401455.
- Watanabe, A.; Gold, M. E. L. G.; Brusatte, S. L.; Benson, R. B. J.; Choiniere, J.; Davidson, A.; Norell, M. A. (2015). "Vertebral Pneumaticity in the Ornithomimosaur Archaeornithomimus (Dinosauria: Theropoda) Revealed by Computed Tomography Imaging and Reappraisal of Axial Pneumaticity in Ornithomimosauria". PLOS ONE. 10 (12): e0145168. Bibcode:2015PLoSO..1045168W. doi:10.1371/journal.pone.0145168. PMC 4684312. PMID 26682888.
- Lee, Y.-N.; Barsbold, R.; Currie, P. J.; Kobayashi, Y.; Lee, H.-J.; Godefroit, P.; Escuillié, F.; Tsogtbaatar, C. (2014). "Resolving the long-standing enigmas of a giant ornithomimosaur Deinocheirus mirificus". Nature. 515 (7526): 257−260. Bibcode:2014Natur.515..257L. doi:10.1038/nature13874. PMID 25337880. S2CID 2986017.
- Hartman, S.; Mortimer, M.; Wahl, W. R.; Lomax, D. R.; Lippincott, J.; Lovelace, D. M. (2019). "A new paravian dinosaur from the Late Jurassic of North America supports a late acquisition of avian flight". PeerJ. 7: e7247. doi:10.7717/peerj.7247. PMC 6626525. PMID 31333906.
- Serrano-Brañas, C. I.; Espinosa-Chávez, B.; Maccracken, S. A.; Gutiérrez-Blando, C.; de León-Dávila, C.; Ventura, J. F. (2020). "Paraxenisaurus normalensis, a large deinocheirid ornithomimosaur from the Cerro del Pueblo Formation (Upper Cretaceous), Coahuila, Mexico". Journal of South American Earth Sciences. 101: 102610. Bibcode:2020JSAES.101j2610S. doi:10.1016/j.jsames.2020.102610.
- Schmitz, L.; Motani, R. (2011). "Nocturnality in Dinosaurs Inferred from Scleral Ring and Orbit Morphology". Science. 332 (6030): 705−708. Bibcode:2011Sci...332..705S. doi:10.1126/science.1200043. PMID 21493820. S2CID 33253407.
- Hall, M. I.; Kirk, E. C.; Kamilar, J. M.; Carrano, M. T. (2011). "Comment on Nocturnality in Dinosaurs Inferred from Scleral Ring and Orbit Morphology". Science. 334 (6063): 1641. Bibcode:2011Sci...334.1641H. doi:10.1126/science.1208442. PMID 22194558.
- Schmitz, L.; Motani, R. (2011). "Response to Comment on Nocturnality in Dinosaurs Inferred from Scleral Ring and Orbit Morphology". Science. 334 (6063): 1641. Bibcode:2011Sci...334.1641S. doi:10.1126/science.1208489.
- Hughes, G. M.; Finarelli, J. A. (2019). "Olfactory receptor repertoire size in dinosaurs". Proceedings of the Royal Society B. 286 (1904): 20190909. doi:10.1098/rspb.2019.0909. PMC 6571463. PMID 31185870.
- Barrett, P. M. (2005). "The diet of ostrich dinosaurs (Theropoda: Ornithomimosauria)". Palaeontology. 48 (2): 347−358. doi:10.1111/j.1475-4983.2005.00448.x.
- Button, D. J.; Zanno, L. E. (2019). "Repeated Evolution of Divergent Modes of Herbivory in Non-avian Dinosaurs". Current Biology. 30 (1): 158−168.e4. doi:10.1016/j.cub.2019.10.050. PMID 31813611. S2CID 208652510.
- Nabavizadeh, A. (2019). "Cranial musculature in herbivorous dinosaurs: a survey of reconstructed anatomical diversity and feeding mechanisms". The Anatomical Record. 303 (4): 1104−1145. doi:10.1002/ar.24283. PMID 31675182. S2CID 207815224.
- Jerzykiewicz, T.; Russell, D. A. (1991). "Late Mesozoic stratigraphy and vertebrates of the Gobi Basin". Cretaceous Research. 12 (4): 345–377. doi:10.1016/0195-6671(91)90015-5.
- Hicks, J. F.; Brinkman, D. L.; Nichols, D. J.; Watabe, M. (1999). "Paleomagnetic and palynologic analyses of Albian to Santonian strata at Bayn Shireh, Burkhant, and Khuren Dukh, eastern Gobi Desert, Mongolia". Cretaceous Research. 20 (6): 829−850. doi:10.1006/cres.1999.0188.
- Ishigaki, S.; Tsogtbaatar, K.; Saneyoshi, M.; Mainbayar, B.; Aoki, K.; Ulziitseren, S.; Imayama, T.; Takahashi, A.; Toyoda, S.; Bayardorj, C.; Buyantegsh, B.; Batsukh, J.; Purevsuren, B.; Asai, H.; Tsutanaga, S.; Fujii, K. (2016). "Report of the Okayama University of Science - Mongolian Institute of Paleontology and Geology Joint Expedition in 2016" (PDF). Bulletin of Research Institute of Natural Sciences (42): 33−46.
- Kurumada, Y.; Aoki, S.; Aoki, K.; Kato, D.; Saneyoshi, M.; Tsogtbaatar, K.; Windley, B. F.; Ishigaki, S. (2020). "Calcite U–Pb age of the Cretaceous vertebrate‐bearing Bayn Shire Formation in the Eastern Gobi Desert of Mongolia: usefulness of caliche for age determination". Terra Nova. 32 (4): 246−252. Bibcode:2020TeNov..32..246K. doi:10.1111/ter.12456.
- Danilov, I. G.; Hirayama, R.; Sukhanov, V. B.; Suzuki, S.; Watabe, M.; Vitek, N. S. (2014). "Cretaceous soft-shelled turtles (Trionychidae) of Mongolia: new diversity, records and a revision". Journal of Systematic Palaeontology. 12 (7): 799–832. doi:10.1080/14772019.2013.847870. S2CID 86304259.
- Lee, Y. M.; Lee, H. J.; Kobayashi, Y.; Carabajal, A. P.; Barsbold, R.; Fiorillo, A. R.; Tsogtbaatar, K. (2019). "Unusual locomotion behaviour preserved within a crocodyliform trackway from the Upper Cretaceous Bayanshiree Formation of Mongolia and its palaeobiological implications". Palaeogeography, Palaeoclimatology, Palaeoecology. 533 (109353): 2. Bibcode:2019PPP...533j9239L. doi:10.1016/j.palaeo.2019.109239.
- Averianov, A.; Sues, H. (2012). "Correlation of Late Cretaceous continental vertebrate assemblages in Middle and Central Asia" (PDF). Journal of Stratigraphy. 36 (2): 462−485. S2CID 54210424.
- Guo, Z. X.; Shi, Y. P.; Yang, Y. T.; Jiang, S. Q.; Li, L. B.; Zhao, Z. G. (2018). "Inversion of the Erlian Basin (NE China) in the early Late Cretaceous: Implications for the collision of the Okhotomorsk Block with East Asia" (PDF). Journal of Asian Earth Sciences. 154: 49−66. Bibcode:2018JAESc.154...49G. doi:10.1016/j.jseaes.2017.12.007.
- Tsogtbaatar, K.; Weishampel, D. B.; Evans, D. C.; Watabe, M. (2019). "A new hadrosauroid (Dinosauria: Ornithopoda) from the Late Cretaceous Baynshire Formation of the Gobi Desert (Mongolia)". PLOS ONE. 14 (4): e0208480. Bibcode:2019PLoSO..1408480T. doi:10.1371/journal.pone.0208480. PMC 6469754. PMID 30995236.
- Park, J. Y.; Lee, Y. N.; Currie, P. J.; Kobayashi, Y.; Koppelhus, E.; Barsbold, R.; Mateus, O.; Lee, S.; Kim, S. H. (2020). "Additional skulls of Talarurus plicatospineus (Dinosauria: Ankylosauridae) and implications for paleobiogeography and paleoecology of armored dinosaurs". Cretaceous Research. 108: 104340. doi:10.1016/j.cretres.2019.104340.
- Powers, M. A.; Sullivan, C.; Currie, P. J. (2020). "Re-examining ratio based premaxillary and maxillary characters in Eudromaeosauria (Dinosauria: Theropoda): Divergent trends in snout morphology between Asian and North American taxa". Palaeogeography, Palaeoclimatology, Palaeoecology. 547 (109704): 109704. Bibcode:2020PPP...547j9704P. doi:10.1016/j.palaeo.2020.109704.
- Zanno, L. E.; Tsogtbaatar, K.; Chinzorig, T.; Gates, T. A. (2016). "Specializations of the mandibular anatomy and dentition of Segnosaurus galbinensis (Theropoda: Therizinosauria)". PeerJ. 4: e1885. doi:10.7717/peerj.1885. PMC 4824891. PMID 27069815.
- Paul, G. S. (2016). The Princeton Field Guide to Dinosaurs (2nd ed.). Princeton, New Jersey: Princeton University Press. pp. 223−224. ISBN 9780691167664.
- Slowiak, J.; Szczygielski, T.; Ginter, M.; Fostowicz-Frelik, L. (2020). "Uninterrupted growth in a non‐polar hadrosaur explains the gigantism among duck‐billed dinosaurs". Palaeontology. 63 (4): 579–599. doi:10.1111/pala.12473.
- Watabe, M.; Suzuki, S. (2000). "Report on the Japan - Mongolia Joint Paleontological Expedition to the Gobi desert, 1993". Hayashibara Museum of Natural Sciences Research Bulletin. 1: 19−29.
- Watabe, M.; Suzuki, S. (2000). "Report on the Japan - Mongolia Joint Paleontological Expedition to the Gobi desert, 1994". Hayashibara Museum of Natural Sciences Research Bulletin. 1: 30−44.
- Kobayashi, Y.; Barsbold, R. (2006). "Ornithomimids from the Nemegt Formation of Mongolia" (PDF). Journal of the Paleontological Society of Korea. 22 (1): 195−207.
External links
- 3D holotype skull at Phenome10k
- 3D reconstructed holotype skull at Phenome10k
- Mounted holotype cast at Youtube