COVID-19 drug development
COVID‑19 drug development is the research process to develop preventative therapeutic prescription drugs that would alleviate the severity of coronavirus disease 2019 (COVID‑19). From early 2020 through 2021, several hundred drug companies, biotechnology firms, university research groups, and health organizations were developing over 600 potential therapies for COVID‑19 disease in various stages of preclinical or clinical research,[1] with 391 therapeutic candidates in clinical trials, as of January 2021.[2]
Part of a series on the |
COVID-19 pandemic |
---|
![]() |
|
![]() |
As early as March 2020, the World Health Organization (WHO),[3] European Medicines Agency (EMA),[4] US Food and Drug Administration (FDA),[5] and the Chinese government and drug manufacturers[6][7] were coordinating with academic and industry researchers to speed development of vaccines, antiviral drugs, and post-infection therapies.[8][9][10][11] The International Clinical Trials Registry Platform of the WHO recorded 536 clinical studies to develop post-infection therapies for COVID‑19 infections,[12][13] with numerous established antiviral compounds for treating other infections under clinical research to be repurposed.[8][14][15][16][17]
In March 2020, the WHO initiated the "SOLIDARITY Trial" in 10 countries, enrolling thousands of people infected with COVID‑19 to assess treatment effects of four existing antiviral compounds with the most promise of efficacy.[3][18] A dynamic, systematic review was established in April 2020 to track the progress of registered clinical trials for COVID‑19 vaccine and therapeutic drug candidates.[13]
Drug development is a multistep process, typically requiring more than five years to assure safety and efficacy of the new compound.[19] Several national regulatory agencies, such as the EMA and the FDA, approved procedures to expedite clinical testing.[5][20] By January 2021, dozens of potential post-infection therapies were in the final stage of human testing – Phase III–IV clinical trials.[1][2][21]
Process
Drug development is the process of bringing a new infectious disease vaccine or therapeutic drug to the market once a lead compound has been identified through the process of drug discovery.[19] It includes laboratory research on microorganisms and animals, filing for regulatory status, such as via the FDA, for an investigational new drug to initiate clinical trials on humans, and may include the step of obtaining regulatory approval with a new drug application to market the drug.[22][23] The entire process – from concept through preclinical testing in the laboratory to clinical trial development, including Phase I–III trials – to approved vaccine or drug typically takes more than a decade.[19][22][23]
New chemical entities
Development of a COVID‑19 vaccine or therapeutic antiviral drug begins with matching a chemical concept to the potential prophylactic mechanism of the future vaccine or antiviral activity in vivo.[22][23][24]
Drug design and laboratory testing
New chemical entities (NCEs, also known as new molecular entities or NMEs) are compounds that emerge from the process of drug discovery to specify a vaccine or antiviral therapeutic candidate having promise for activity against a biological target related to COVID‑19 disease.[26] At the beginning of vaccine or drug development, little is known about the safety, toxicity, pharmacokinetics, and metabolism of the NCE in humans.[19][22][23] It is the function and obligation of drug development to assess all of these parameters prior to human clinical trials to prove safety and efficacy. A further major objective of drug development is to recommend the dose and schedule for the first use in a human clinical trial ("first-in-human" [FIH] or First Human Dose [FHD], previously also known as "first-in-man" [FIM]).
In addition, drug development must establish the physicochemical properties of the NCE: its chemical makeup, stability, and solubility. Manufacturers must optimize the process they use to make the chemical so they can scale up from a medicinal chemist producing milligrams, to manufacturing on the kilogram and ton scale.[22][23] They further examine the product for suitability to package as capsules, tablets, aerosol, intramuscular injectable, subcutaneous injectable, or intravenous formulations. Together, these processes are known in preclinical and clinical development as chemistry, manufacturing, and control (CMC).[22]
Many aspects of drug development focus on satisfying the regulatory requirements of drug licensing authorities.[19] These generally constitute tests designed to determine the major toxicities of a novel compound prior to first use in humans.[19][22] It is a regulatory requirement that an assessment of major organ toxicity be performed (effects on the heart and lungs, brain, kidney, liver and digestive system), as well as effects on other parts of the body that might be affected by the drug (e.g., the skin if the new vaccine is to be delivered by skin injection). Increasingly, these tests are made using in vitro methods (e.g., with isolated cells), but many tests can only be made by using experimental animals to demonstrate the complex interplay of metabolism and drug exposure on toxicity.[22][27]
The information is gathered from this preclinical testing, as well as information on CMC, and submitted to regulatory authorities (in the US, to the Food and Drug Administration (FDA)), as an Investigational New Drug (IND) or Biologics License Application (BLA) application for a vaccine.[19][22][23][24] If the IND is approved, development moves to the clinical phase,[19] and the progress of performance in humans – if a vaccine under development in the United States – is monitored by the FDA in a "vaccine approval process."[28]
Efforts to streamline drug discovery
Over 2018–20, new initiatives to stimulate vaccine and antiviral drug development included partnerships between governmental organizations and industry, such as the European Innovative Medicines Initiative,[29] the US Critical Path Initiative to enhance innovation of drug development,[30] and the Breakthrough Therapy designation to expedite development and regulatory review of promising candidate drugs.[31] To accelerate refinement of diagnostics for detecting COVID‑19 infection, a global diagnostic pipeline tracker was formed.[32]
During March 2020, the Coalition for Epidemic Preparedness Innovations (CEPI) initiated an international COVID‑19 vaccine development fund, with the goal to raise US$2 billion for vaccine research and development,[33] and committed to investments of US$100 million in vaccine development across several countries.[34] The Canadian government announced CA$275 million in funding for 96 research projects on medical countermeasures against COVID‑19, including numerous vaccine candidates at Canadian universities,[35][36] with plans to establish a "vaccine bank" of new vaccines for implementation if another coronavirus outbreak occurs.[36][37] The Bill & Melinda Gates Foundation invested US$150 million in April for development of COVID‑19 vaccines, diagnostics, and therapeutics.[38]
Computer-assisted research
In March 2020, the United States Department of Energy, National Science Foundation, NASA, industry, and nine universities pooled resources to access supercomputers from IBM, combined with cloud computing resources from Hewlett Packard Enterprise, Amazon, Microsoft, and Google, for drug discovery.[39][40] The COVID‑19 High Performance Computing Consortium also aims to forecast disease spread, model possible vaccines, and screen thousands of chemical compounds to design a COVID‑19 vaccine or therapy.[39][40]
The C3.ai Digital Transformation Institute, an additional consortium of Microsoft, six universities (including the Massachusetts Institute of Technology, a member of the first consortium), and the National Center for Supercomputer Applications in Illinois, working under the auspices of C3.ai, an artificial intelligence software company, are pooling supercomputer resources toward drug discovery, medical protocol development and public health strategy improvement, as well as awarding large grants to researchers who proposed by May to use AI to carry out similar tasks.[41][42]
In March 2020, the distributed computing project Folding@home launched a program to assist drug developers, initially simulating protein targets from SARS-CoV-2 and the related SARS-CoV virus, which has been studied previously.[43][44][45]
Distributed computing project Rosetta@home also joined the effort in March. The project uses computers of volunteers to model SARS-CoV-2 virus proteins to discover possible drug targets or create new proteins to neutralize the virus. Researchers revealed that with the help of Rosetta@home, they had been able to “accurately predict the atomic-scale structure of an important coronavirus protein weeks before it could be measured in the lab.”[46]
In May 2020, the OpenPandemics – COVID‑19 partnership between Scripps Research and IBM's World Community Grid was launched. The partnership is a distributed computing project that "will automatically run a simulated experiment in the background [of connected home PCs] which will help predict the effectiveness of a particular chemical compound as a possible treatment for COVID‑19".[47]
Clinical trial phases
Clinical trial programs involve three, multiple-year stages toward product approval, and a fourth, post-approval stage for ongoing safety monitoring of the vaccine or drug therapy:[19][48]
- Phase I trials, usually in healthy volunteers, determine safety and dosing.
- Phase II trials are used to establish an initial reading of efficacy and further explore safety in small numbers of people having the disease targeted by the NCE.
- Phase III trials are large, pivotal trials to determine safety and efficacy in sufficiently large numbers of people with the COVID‑19 infection. If safety and efficacy are adequately proved, clinical testing may stop at this step and the NCE advances to the new drug application (NDA) stage to begin marketing.[19]
- Phase IV trials are post-approval trials that may be a condition attached by the FDA, also called post-market surveillance studies. Until a vaccine is provided to the general population, all potential adverse events remain unidentified, requiring that vaccines undergo Phase IV studies with regular reports by the manufacturer to the Vaccine Adverse Event Reporting System (VAERS) to identify problems after use in the population begins.[28]
The process of defining characteristics of the drug does not stop once an NCE is advanced into human clinical trials. In addition to the tests required to move a novel vaccine or antiviral drug into the clinic for the first time, manufacturers must ensure that any long-term or chronic toxicities are well-defined, including effects on systems not previously monitored (fertility, reproduction, immune system, among others).[22][28] If a vaccine candidate or antiviral compound emerges from these tests with an acceptable toxicity and safety profile, and the manufacturer can further show it has the desired effect in clinical trials, then the NCE portfolio of evidence can be submitted for marketing approval in the various countries where the manufacturer plans to sell it.[19] In the United States, this process is called a "new drug application" or NDA.[19][22]
Adaptive designs for COVID‑19 trials
A clinical trial design in progress may be modified as an "adaptive design" if accumulating data in the trial provide early insights about positive or negative efficacy of the treatment.[49][50] The global Solidarity and European Discovery trials of hospitalized people with severe COVID‑19 infection apply adaptive design to rapidly alter trial parameters as results from the four experimental therapeutic strategies emerge.[12][51][52] Adaptive designs within ongoing Phase II–III clinical trials on candidate therapeutics may shorten trial durations and use fewer subjects, possibly expediting decisions for early termination or success, and coordinating design changes for a specific trial across its international locations.[50][53][54]
Failure rate
Most novel drug candidates (NCEs) fail during drug development, either because they have unacceptable toxicity or because they simply do not prove efficacy on the targeted disease, as shown in Phase II–III clinical trials.[19][22] Critical reviews of drug development programs indicate that Phase II–III clinical trials fail due mainly to unknown toxic side effects (50% failure of Phase II cardiology trials), and because of inadequate financing, trial design weaknesses, or poor trial execution.[53][55]
A study covering clinical research in the 1980–90s found that only 21.5% of drug candidates that started Phase I trials were eventually approved for marketing.[56] During 2006–15, the success rate of obtaining approval from Phase I to successful Phase III trials was under 10% on average, and 16% specifically for vaccines.[57] The high failure rates associated with pharmaceutical development are referred to as an "attrition rate", requiring decisions during the early stages of drug development to "kill" projects early to avoid costly failures.[57][58]
Cost
One 2010 study assessed both capitalized and out-of-pocket costs for bringing a single new drug to market as about US$1.8 billion and $870 million, respectively.[59] A median cost estimate of 2015–16 trials for development of 10 anti-cancer drugs was $648 million.[60] In 2017, the median cost of a pivotal trial across all clinical indications was $19 million.[61]
The average cost (2013 dollars) of each stage of clinical research was US$25 million for a Phase I safety study, $59 million for a Phase II randomized controlled efficacy study, and $255 million for a pivotal Phase III trial to demonstrate its equivalence or superiority to an existing approved drug,[62] possibly as high as $345 million.[61] The average cost of conducting a 2015–16 pivotal Phase III trial on an infectious disease drug candidate was $22 million.[61]
The full cost of bringing a new drug (i.e., new chemical entity) to market – from discovery through clinical trials to approval – is complex and controversial.[22][23][61][63] In a 2016 review of 106 drug candidates assessed through clinical trials, the total capital expenditure for a manufacturer having a drug approved through successful Phase III trials was $2.6 billion (in 2013 dollars), an amount increasing at an annual rate of 8.5%.[62] Over 2003–2013 for companies that approved 8–13 drugs, the cost per drug could rise to as high as $5.5 billion, due mainly to international geographic expansion for marketing and ongoing costs for Phase IV trials for continuous safety surveillance.[64]
Alternatives to conventional drug development have the objective for universities, governments, and the pharmaceutical industry to collaborate and optimize resources.[65]
COVID-19 clinical trials overview: timelines in 2020
According to two organizations tracking clinical trial progress on potential therapeutic drugs for COVID‑19 infections, 29 Phase II–IV efficacy trials were concluded in March or scheduled to provide results in April from hospitals in China – which experienced the first outbreak of COVID‑19 in late 2019.[1][21] Seven trials were evaluating repurposed drugs already approved to treat malaria, including four studies on hydroxychloroquine or chloroquine phosphate.[21] Repurposed antiviral drugs make up most of the Chinese research, with 9 Phase III trials on remdesivir across several countries due to report by the end of April.[21] Other potential therapeutic candidates under pivotal clinical trials concluding in March–April are vasodilators, corticosteroids, immune therapies, lipoic acid, bevacizumab, and recombinant angiotensin-converting enzyme 2, among others.
The COVID‑19 Clinical Research Coalition has goals to 1) facilitate rapid reviews of clinical trial proposals by ethics committees and national regulatory agencies, 2) fast-track approvals for the candidate therapeutic compounds, 3) ensure standardised and rapid analysis of emerging efficacy and safety data, and 4) facilitate sharing of clinical trial outcomes before publication.[12] A dynamic review of clinical development for COVID‑19 vaccine and drug candidates was in place, as of April.[13]
By March 2020, the international Coalition for Epidemic Preparedness Innovations (CEPI) committed to research investments of US$100 million across several countries,[34] and issued an urgent call to raise and rapidly invest $2 billion for vaccine development.[66] Led by the Bill and Melinda Gates Foundation with partners investing US$125 million and coordinating with the World Health Organization, the COVID‑19 Therapeutics Accelerator began in March, facilitating drug development researchers to rapidly identify, assess, develop, and scale up potential treatments.[67] The COVID‑19 Clinical Research Coalition formed to coordinate and expedite results from international clinical trials on the most promising post-infection treatments.[12] In early 2020, numerous established antiviral compounds for treating other infections were being repurposed or developed in new clinical research efforts to alleviate the illness of COVID‑19.[8][14][15][21]
Therapeutic candidates
Phase III–IV trials
Pivotal Phase III trials assess whether a candidate drug has efficacy specifically against a disease, and – in the case of people hospitalized with severe COVID‑19 infections – test for an effective dose level of the repurposed or new drug candidate to improve the illness (primarily pneumonia) from COVID‑19 infection.[3][12][69] For an already-approved drug (such as hydroxychloroquine for malaria), Phase III–IV trials determine in hundreds to thousands of COVID‑19-infected people the possible extended use of an already-approved drug for treating COVID‑19 infection.[69] As of August 2020, over 500 candidate therapeutics were in preclinical or a stage of Phase I–IV development,[1] with new Phase II–III trials announced for hundreds of therapeutic candidates during 2020.[21][1][2]
International Solidarity and Discovery Trials
In March, the World Health Organization (WHO) launched the coordinated "Solidarity Trial" in 10 countries on five continents to rapidly assess in thousands of COVID‑19 infected people the potential efficacy of existing antiviral and anti-inflammatory agents not yet evaluated specifically for COVID‑19 illness.[3][18] By late April, hospitals in over 100 countries were involved in the trial.[70]
The individual or combined drugs undergoing initial studied are 1) lopinavir–ritonavir combined, 2) lopinavir–ritonavir combined with interferon-beta, 3) remdesivir or 4) (hydroxy)chloroquine in separate trials and hospital sites internationally.[3][18] Following a study published by The Lancet on safety concerns with hydroxychloroquine, the WHO suspended use of it from the Solidarity trial in May 2020,[71][72] reinstated it after the research was retracted,[73] then abandoned further use of the drug for COVID‑19 treatment when analysis showed in June that it provided no benefit.[74]
With about 15% of people infected by COVID‑19 having severe illness, and hospitals being overwhelmed during the pandemic, WHO recognized a rapid clinical need to test and repurpose these drugs as agents already approved for other diseases and recognized as safe.[3] The Solidarity project is designed to give rapid insights to key clinical questions:[3][75]
- Do any of the drugs reduce mortality?
- Do any of the drugs reduce the time a patient is hospitalized?
- Do the treatments affect the need for people with COVID‑19-induced pneumonia to be ventilated or maintained in intensive care?
- Could such drugs be used to minimize the illness of COVID‑19 infection in healthcare staff and people at high risk of developing severe illness?
Enrolling people with COVID‑19 infection is simplified by using data entries, including informed consent, on a WHO website.[3] After the trial staff determines the drugs available at the hospital, the WHO website randomizes the hospitalized subject to one of the trial drugs or to the hospital standard of care for treating COVID‑19. The trial physician records and submits follow-up information about the subject status and treatment, completing data input via the WHO Solidarity website.[3] The design of the Solidarity trial is not double-blind – which is normally the standard in a high-quality clinical trial – but the WHO needed speed with quality for the trial across many hospitals and countries.[3] A global safety monitoring board of WHO physicians examine interim results to assist decisions on safety and effectiveness of the trial drugs, and alter the trial design or recommend an effective therapy.[3][75] A similar web-based study to Solidarity, called "Discovery", was initiated in March across seven countries by INSERM (Paris, France).[3][51]
The Solidarity trial seeks to implement coordination across hundreds of hospital sites in different countries – including those with poorly-developed infrastructure for clinical trials – yet needs to be conducted rapidly. According to John-Arne Røttingen, chief executive of the Research Council of Norway and chairman of the Solidarity trial international steering committee, the trial would be considered effective if therapies are determined to "reduce the proportion of patients that need ventilators by, say, 20%, that could have a huge impact on our national health-care systems."[76]
During March, funding for the Solidarity trial reached US$108 million from 203,000 individuals, organizations and governments, with 45 countries involved in financing or trial management.[77]
Recovery Trial
During April, the British RECOVERY (Randomised Evaluation of COVid-19 thERapY) trial was launched initially in 132 hospitals across the UK,[78] expanding to become one of the world's largest COVID‑19 clinical studies, involving 5400 infected people under treatment at 165 UK hospitals, as of mid-April.[79] The trial is examining different potential therapies for severe COVID‑19 infection: lopinavir/ritonavir, low-dose dexamethasone (an anti-inflammatory steroid), hydroxychloroquine, and azithromycin (a common antibiotic).[80] In June, the trial arm using hydroxychloroquine was discontinued when analyses showed it provided no benefit.[81]
On 16 June the trial group released a statement that dexamethasone had been shown to reduce mortality in patients receiving respiratory support.[82] In a controlled trial around 2,000 hospital patients were given dexamethasone and were compared with more than 4,000 who did not receive the drug. For patients on ventilators, it cut the risk of death from 40% to 28% (1 in 8). For patients needing oxygen, it cut the risk of death from 25% to 20% (1 in 5).[83]
Adaptive COVID-19 Treatment Trial
The US National Institute of Allergy and Infectious Diseases (NIAID) initiated an adaptive design, international Phase III trial (called "ACTT") to involve up to 800 hospitalized COVID‑19 people at 100 sites in multiple countries.[84] Beginning with use of remdesivir as the primary treatment over 29 days, the trial definition of its adaptive protocol states that "there will be interim monitoring to introduce new arms and allow early stopping for futility, efficacy, or safety. If one therapy proves to be efficacious, then this treatment may become the control arm for comparison(s) with new experimental treatment(s)."[84]
Tabulating late-stage treatment candidates
Numerous candidate drugs under study as "supportive" treatments to relieve discomfort during illness, such as NSAIDs or bronchodilators, are not included in the table below. Others in early-stage Phase II trials or numerous treatment candidates in Phase I trials,[21][1] are also excluded. Drug candidates in Phase I–II trials have a low rate of success (under 12%) to pass through all trial phases to gain eventual approval.[22][53] Once having reached Phase III trials, therapeutic candidates for diseases related to COVID‑19 infection – infectious and respiratory diseases – have a success rate of about 72%.[57]
COVID‑19: candidate drug treatments in Phase III–IV trials | ||||||
---|---|---|---|---|---|---|
Drug candidate | Description | Existing disease approval | Trial sponsor(s) | Location(s) | Expected results | Notes, references |
Remdesivir | antiviral; adenosine nucleotide analog inhibiting RNA synthesis in coronaviruses | investigational[85] | Gilead, WHO, INSERM, NIAID | China, Japan initially; extended internationally in Global Solidarity and Discovery Trials, and US NIAID ACTT Trial | Mid-2020 (Chinese, Japanese trials) | [21][51][86] selectively provided by Gilead for COVID‑19 emergency access;[87][88] both promising and negative effects reported in April[84][89][90] |
Hydroxychloroquine or chloroquine | antiparasitic and antirheumatic; generic made by many manufacturers | malaria, rheumatoid arthritis, lupus (International)[91][92] | CEPI, WHO, INSERM | Multiple sites in China; global Solidarity and Discovery Trials | June 2020 (discontinued by WHO) | multiple side effects; possible adverse prescription drug interactions;[91][92] discontinued in June from WHO Solidarity trial and UK Recovery trial as "having no clinical benefit in hospitalised patients with COVID‑19";[74][81] trials[21][51] |
Favipiravir | antiviral against influenza | influenza (China)[93] | Fujifilm | China | April 2020 | [21][9][94] |
Lopinavir/ritonavir without or with interferon beta-1a | antiviral, immune suppression | investigational combination; lopinavir/ritonavir approved[95] | CEPI, WHO, UK Government, Univ. of Oxford, INSERM | Global Solidarity and Discovery Trials, multiple countries | mid-2020 | [21][51] |
Sarilumab | human monoclonal antibody against interleukin-6 receptor | rheumatoid arthritis (US, Europe)[96] | Regeneron-Sanofi | Multiple countries | Spring 2020 | [21][97] |
ASC-09 + ritonavir | antiviral | combination not approved; ritonavir approved for HIV[95] | Ascletis Pharma | Multiple sites in China | Spring 2020 | [21][98] |
Tocilizumab | human monoclonal antibody against interleukin-6 receptor | immunosuppression, rheumatoid arthritis (US, Europe)[99] | Genentech-Hoffmann-La Roche | Multiple countries | mid-2020 | [21][1][100] Roche announced in late July that its Phase III trial of tocilizumab for treating pneumonia in hospitalized people with COVID-19 infection was ineffective[101] |
Lenzilumab | humanized monoclonal antibody for relieving pneumonia | new drug candidate | Humanigen, Inc. | Multiple sites in the United States | September 2020 | [21][102] |
Dapagliflozin | sodium-glucose cotransporter 2 inhibitor | hypoglycemia agent[103] | Saint Luke's Mid America Heart Institute, AstraZeneca | Multiple countries | December 2020 | [21][104] |
CD24Fc | antiviral immunomodulator against inflammatory response | new drug candidate | OncoImmune, Inc. | Multiple sites in the United States | 2021 | [21][105] |
Chloroquine and hydroxychloroquine
Chloroquine is an anti-malarial medication that is also used against some auto-immune diseases. Hydroxychloroquine is more commonly available than chloroquine in the United States.[88] Although several countries initially used chloroquine or hydroxychloroquine for treatment of persons hospitalized with COVID‑19 (as of March 2020), the drug has not been formally approved through clinical trials,[88][106] and its use was withdrawn as a possible treatment for COVID‑19 infection when it proved to have no benefit for hospitalized patients with severe COVID-19 illness in the international Solidarity trial and UK RECOVERY Trial.[74][81]
In the United States, the experimental treatment was first authorized only for emergency use for people hospitalized but unable to receive treatment in a clinical trial,[107] but this authorization was revoked by the FDA in June, stating that it was "no longer reasonable to believe" that the drug was effective against COVID‑19 or that its benefits outweighed "known and potential risks".[108] Hydroxychloroquine is used as a prophylactic in India.[109][110]
In November 2020, a US National Institutes of Health (NIH) clinical trial evaluating the safety and effectiveness of hydroxychloroquine for the treatment of adults with coronavirus disease 2019 (COVID-19) has formally concluded that the drug provides no clinical benefit to hospitalized patients.[111][112]
Background
Chloroquine was initially recommended by Indian, Chinese, South Korean and Italian health authorities for the treatment of COVID‑19,[113] although these agencies and the US CDC noted contraindications for people with heart disease or diabetes.[88][114] In February 2020, both drugs were shown to effectively reduce COVID‑19 illness, but a further study concluded that hydroxychloroquine was more potent than chloroquine and had a more tolerable safety profile.[115][116] On 18 March, the WHO announced that chloroquine and the related hydroxychloroquine would be among the four drugs studied as part of the Solidarity clinical trial.[117]
Hydroxychloroquine and chloroquine have numerous, potentially serious, side effects, such as retinopathy, hypoglycemia, or life-threatening arrhythmia and cardiomyopathy.[91] Both drugs have extensive interactions with prescription drugs, affecting the therapeutic dose and disease mitigation.[91][92] Some people have allergic reactions to these drugs.[91][92] The NIH recommended against the use of a combination of hydroxychloroquine and azithromycin because of the resulting increased risk of sudden cardiac death.[118]
Favipiravir
Chinese clinical trials in Wuhan and Shenzhen claimed to show that favipiravir was "clearly effective".[119] Of 35 patients in Shenzhen tested negative in a median of 4 days, while the length of illness was 11 days in the 45 patients who did not receive it.[120] In a study conducted in Wuhan on 240 patients with pneumonia half were given favipiravir and half received umifenovir. The researchers found that patients recovered from coughs and fevers faster when treated with favipiravir, but that there was no change in how many patients in each group progressed to more advanced stages of illness that required treatment with a ventilator.[121]
On 22 March 2020, Italy approved the drug for experimental use against COVID‑19 and began conducting trials in the three regions most affected by the disease.[122] The Italian Pharmaceutical Agency reminded the public that the existing evidence in support of the drug is scant and preliminary.[123]
On 30 May 2020, the Russian Health Ministry approved a generic version of favipiravir named Avifavir, which proved highly effective in the first phase of clinical trials.[124][125][126]
In June 2020, India approved the use of a generic version of favipravir called FabiFlu, developed by Glenmark Pharmaceuticals, in the treatment of mild to moderate cases of COVID‑19.[127]
Remdesivir
A nucleotide analog, remdesivir is an antiviral drug candidate originally developed to treat Ebola virus disease.[128] It is specifically an adenosine analog which inserts into viral RNA chains, causing premature breaking of the chains.[129] It was studied as a possible post-infection treatment for COVID-19.[3] In May 2020, there were nine Phase III trials on remdesivir across several countries.[21][85] In November 2020, the World Health Organization updated its guideline on therapeutics for COVID-19 to include a conditional recommendation against the use of remdesivir, triggered by results from the WHO Solidarity trial.[130][131]
Adverse effects
The most common adverse effects in people treated with remdesivir were respiratory failure and blood biomarkers of organ impairment, including low albumin, low potassium, low count of red blood cells, low count of thrombocytes, and elevated bilirubin (jaundice).[90] Other reported adverse effects include gastrointestinal distress, elevated transaminase levels in the blood (liver enzymes), infusion site reactions, and electrocardiogram abnormalities.[132] Remdesivir may cause infusion‐related reactions, including low blood pressure, nausea, vomiting, sweating or shivering.[133]
Casirivimab/imdevimab
On 21 November 2020, the U.S. Food and Drug Administration (FDA) issued an emergency use authorization (EUA) for casirivimab and imdevimab to be administered together for the treatment of mild to moderate COVID‑19 in people twelve years of age or older weighing at least 40 kilograms (88 lb) with positive results of direct SARS‑CoV‑2 viral testing and who are at high risk for progressing to severe COVID‑19.[134] This includes those who are 65 years of age or older or who have certain chronic medical conditions.[134]
On 1 February 2021, the Committee for Medicinal Products for Human Use (CHMP) of the European Medicines Agency (EMA) started a rolling review of data on the REGN‑COV2 antibody combination (casirivimab/imdevimab), which is being co-developed by Regeneron Pharmaceuticals, Inc. and F. Hoffman-La Roche, Ltd (Roche) for the treatment and prevention of COVID‑19.[135]
Strategies
Repurposing approved drugs
Drug repositioning (also called drug repurposing) – the investigation of existing drugs for new therapeutic purposes – is one line of scientific research followed to develop safe and effective COVID‑19 treatments.[17][136] Several existing antiviral medications, previously developed or used as treatments for Severe acute respiratory syndrome (SARS), Middle East respiratory syndrome (MERS), HIV/AIDS, and malaria, are being researched as COVID‑19 treatments, with some moving into clinical trials.[137]
During the COVID‑19 outbreak, drug repurposing is the clinical research process of rapidly screening and defining the safety and efficacy of existing drugs already approved for other diseases to be used for people with COVID‑19 infection.[14][17][138] In the usual drug development process,[19] confirmation of repurposing for new disease treatment would take many years of clinical research – including pivotal Phase III clinical trials – on the candidate drug to assure its safety and efficacy specifically for treating COVID‑19 infection.[14][138] In the emergency of a growing COVID‑19 pandemic, the drug repurposing process was being accelerated during March 2020 to treat people hospitalized with COVID‑19.[3][14][17]
Clinical trials using repurposed, generally safe, existing drugs for hospitalized COVID‑19 people may take less time and have lower overall costs to obtain endpoints proving safety (absence of serious side effects) and post-infection efficacy, and can rapidly access existing drug supply chains for manufacturing and worldwide distribution.[3][14][139] In an international effort to capture these advantages, the WHO began in mid-March 2020 expedited international Phase II–III trials on four promising treatment options – the SOLIDARITY trial[3][140][141] – with numerous other drugs having potential for repurposing in different disease treatment strategies, such as anti-inflammatory, corticosteroid, antibody, immune, and growth factor therapies, among others, being advanced into Phase II or III trials during 2020.[21][14][15][138][142]
In March, the United States Centers for Disease Control and Prevention (CDC) issued a physician advisory concerning remdesivir for people hospitalized with pneumonia caused by COVID‑19: "While clinical trials are critical to establish the safety and efficacy of this drug, clinicians without access to a clinical trial may request remdesivir for compassionate use through the manufacturer for patients with clinical pneumonia."[88]
Preliminary clinical research: Phase II trials
Phase I trials test primarily for safety and preliminary dosing in a few dozen healthy subjects, while Phase II trials – following success in Phase I – evaluate therapeutic efficacy against the COVID‑19 disease at ascending dose levels (efficacy based on biomarkers), while closely evaluating possible adverse effects of the candidate therapy (or combined therapies), typically in hundreds of people.[143] A common trial design for Phase II studies of possible COVID‑19 drugs is randomized, placebo-controlled, blinded, and conducted at multiple sites, while determining more precise, effective doses and monitoring for adverse effects.[143]
The success rate for Phase II trials to advance to Phase III (for all diseases) is about 31%, and for infectious diseases specifically, about 43%.[57] Depending on its duration (longer more expensive) – typically a period of several months to two years[143] – an average-length Phase II trial costs US$57 million (2013 dollars, including preclinical and Phase I costs).[62] Successful completion of a Phase II trial does not reliably forecast that a candidate drug will be successful in Phase III research.[53]
Phase III trials for COVID‑19 involve hundreds-to-thousands of hospitalized participants, and test effectiveness of the treatment to reduce effects of the disease, while monitoring for adverse effects at the optimal dose, such as in the multinational Solidarity and Discovery trials.[76][3][19]
On 13 October 2020, a Phase II-III trial on a candidate treatment using a monoclonal antibody technology developed by AbCellera Biologics and Eli Lilly, bamlanivimab (LY-CoV555), was paused due to safety concerns.[144][145][146]
On 26 October 2020, Eli Lilly announced that the National Institutes of Health (NIH) ACTIV-3 clinical trial evaluating its monoclonal antibody, bamlanivimab (LYCoV555), found that bamlanivimab was not effective in treating people hospitalized with COVID-19.[147] Other studies, including the NIH ACTIV-2 trial and its own BLAZE-1 trial, will continue to evaluate bamlanivimab.[147]
According to a source reporting early-stage clinical trials on potential COVID‑19 post-infection therapies, there were over 230 Phase II trials underway or planned to start by October 2020.[2]
Emergency use authorization
On 7 October 2020, Eli Lilly and Company submitted a request for an Emergency Use Authorization (EUA) to the U.S. Food and Drug Administration (FDA) for LY-CoV555 monotherapy in higher-risk people who have been diagnosed with mild-to-moderate COVID-19.[148] Bamlanivimab (LY-CoV555) is a neutralizing IgG1 monoclonal antibody (mAb) directed against the spike protein of SARS-CoV-2.[148] It is being tested in the BLAZE-1, BLAZE-2, and ACTIV-3 trials.[148]
On 9 November 2020, the U.S. Food and Drug Administration issued an emergency use authorization for the investigational monoclonal antibody therapy bamlanivimab for the treatment of mild-to-moderate COVID-19.[149] Bamlanivimab is authorized for people with positive results of direct SARS-CoV-2 viral testing who are twelve years of age and older weighing at least 40 kilograms (88 lb), and who are at high risk for progressing to severe COVID-19 or hospitalization.[149] This includes those who are 65 years of age or older, or who have certain chronic medical conditions.[149]
Categories of potential therapeutics against COVID-19
According to one source (as of August 2020), diverse categories of preclinical or early-stage clinical research for developing COVID‑19 therapeutic candidates included:[21]
- antibodies (81 candidates)
- antivirals (31 candidates)
- cell-based compounds (34 candidates)
- RNA-based compounds (6 candidates)
- scanning compounds to be repurposed (18 candidates)
- various other therapy categories, such as anti-inflammatory, antimalarial, interferon, protein-based, antibiotics, and receptor-modulating compounds, among others for a total of some 511 compounds under development in August.[1]
Protease inhibitors
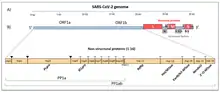
In March 2020, the main protease (3CLpro) of the SARS-CoV-2 virus was identified as a target for post-infection drugs. The enzyme is essential for processing the replication-related polyprotein. To find the enzyme, scientists used the genome published by Chinese researchers in January 2020 to isolate the main protease.[150] Protease inhibitors approved for treating human immunodeficiency viruses (HIV) – lopinavir and ritonavir – have preliminary evidence of activity against the coronaviruses, SARS and MERS.[3][14] As a potential combination therapy, they are used together in two Phase III arms of the 2020 global Solidarity project on COVID‑19.[3][76] A preliminary study in China of combined lopinavir and ritonavir found no effect in people hospitalized for COVID‑19.[151] Novel protease inhibitors, which specifically target the protease 3CLpro, are being researched and developed in the laboratory such as CLpro-1, GC376, and Rupintrivir.[152][153][154]
Preclinical research
The term "preclinical research" is defined by laboratory studies in vitro and in vivo, indicating a beginning stage for development of a preventative vaccine, antiviral or other post-infection therapies,[8] such as experiments to determine effective doses and toxicity in animals, before a candidate compound is advanced for safety and efficacy evaluation in humans.[155] To complete the preclinical stage of drug development – then be tested for safety and efficacy in an adequate number of people infected with COVID‑19 (hundreds to thousands in different countries) – is a process likely to require 1–2 years for COVID‑19 therapies, according to several reports in early 2020.[10][156][157][158] Despite these efforts, the success rate for drug candidates to reach eventual regulatory approval through the entire drug development process for treating infectious diseases is only 19%.[57]
Broad-spectrum therapeutics
In 2021, the importance of drug repurposing for COVID-19 led to the establishment of broad-spectrum therapeutics.[159] Broad-spectrum therapeutics are effective against multiple types of pathogens.[160] Such drugs have been suggested as potential emergency treatments for future pandemics.[161][162]
See also
References
- "Biopharma products in development for COVID-19". BioWorld. 2021-01-15. Retrieved 2021-01-18.
- "COVID-19 vaccine and therapeutics tracker". BioRender. 2021-01-15. Retrieved 2021-01-18.
- Kupferschmidt K, Cohen J (22 March 2020). "WHO launches global megatrial of the four most promising coronavirus treatments". Science Magazine. doi:10.1126/science.abb8497. Retrieved 27 March 2020.
- "First regulatory workshop on COVID-19 facilitates global collaboration on vaccine development". European Medicines Agency. 18 March 2020. Retrieved 21 March 2020.
- "Coronavirus (COVID-19) Update: FDA Continues to Facilitate Development of Treatments" (Press release). U.S. Food and Drug Administration (FDA). 19 March 2020. Retrieved 21 March 2020.
- "China approves first anti-viral drug against coronavirus Covid-19". Clinical Trials Arena. 18 February 2020. Retrieved 21 March 2020.
- "Chinese Vaccine Approved for Human Testing at Virus Epicenter". Bloomberg News. 19 March 2020. Retrieved 21 March 2020.
- Dhama K, Sharun K, Tiwari R, Dadar M, Malik YS, Singh KP, Chaicumpa W (June 2020). "COVID-19, an emerging coronavirus infection: advances and prospects in designing and developing vaccines, immunotherapeutics, and therapeutics". Human Vaccines & Immunotherapeutics. 16 (6): 1232–1238. doi:10.1080/21645515.2020.1735227. PMC 7103671. PMID 32186952.
- Zhang L, Liu Y (May 2020). "Potential interventions for novel coronavirus in China: A systematic review". Journal of Medical Virology. 92 (5): 479–490. doi:10.1002/jmv.25707. PMC 7166986. PMID 32052466.
- Fox M (19 March 2020). "Drug makers are racing to develop immune therapies for Covid-19. Will they be ready in time?". Stat. Retrieved 21 March 2020.
- Chan M (19 March 2020). "Chinese military scientists ordered to win global race to develop coronavirus vaccine". South China Morning Post. Retrieved 22 March 2020.
- COVID-19 Clinical Research Coalition (April 2020). "Global coalition to accelerate COVID-19 clinical research in resource-limited settings". Lancet. 395 (10233): 1322–1325. doi:10.1016/s0140-6736(20)30798-4. PMC 7270833. PMID 32247324.
- Maguire BJ, Guérin PJ (2 April 2020). "A living systematic review protocol for COVID-19 clinical trial registrations". Wellcome Open Research. 5: 60. doi:10.12688/wellcomeopenres.15821.1. PMC 7141164. PMID 32292826.
- Li G, De Clercq E (March 2020). "Therapeutic options for the 2019 novel coronavirus (2019-nCoV)". Nature Reviews. Drug Discovery. 19 (3): 149–150. doi:10.1038/d41573-020-00016-0. PMID 32127666.
- Li G, De Clercq E (March 2020). "Therapeutic options for the 2019 novel coronavirus (2019-nCoV)". Nature Reviews. Drug Discovery. 19 (3): 149–150. doi:10.1038/d41573-020-00016-0. PMID 32127666.
- Dong L, Hu S, Gao J (2020-02-29). "Discovering drugs to treat coronavirus disease 2019 (COVID-19)". Drug Discoveries & Therapeutics. 14 (1): 58–60. doi:10.5582/ddt.2020.01012. PMID 32147628.
- Harrison C (April 2020). "Coronavirus puts drug repurposing on the fast track". Nature Biotechnology. 38 (4): 379–381. doi:10.1038/d41587-020-00003-1. PMID 32205870.
- Cheng MP, Lee TC, Tan DH, Murthy S (April 2020). "Generating randomized trial evidence to optimize treatment in the COVID-19 pandemic". Cmaj. 192 (15): E405–E407. doi:10.1503/cmaj.200438. PMC 7162442. PMID 32336678.
- "The Drug Development Process". U.S. Food and Drug Administration (FDA). 4 January 2018. Retrieved 21 March 2020.
- "Call to pool research resources into large multi-centre, multi-arm clinical trials to generate sound evidence on COVID-19 treatments". European Medicines Agency. 19 March 2020. Retrieved 21 March 2020.
- "COVID-19 vaccine and treatments tracker (Choose vaccines or treatments tab, apply filters to view select data)". Milken Institute. 2021-01-14. Retrieved 2021-01-18. Lay summary.
- Strovel J, Sittampalam S, Coussens NP, Hughes M, Inglese J, Kurtz A, et al. (July 1, 2016). "Early Drug Discovery and Development Guidelines: For Academic Researchers, Collaborators, and Start-up Companies". Assay Guidance Manual. Eli Lilly & Company and the National Center for Advancing Translational Sciences. PMID 22553881.
- Taylor D (2015). "The Pharmaceutical Industry and the Future of Drug Development". Issues in Environmental Science and Technology. Royal Society of Chemistry: 1–33. doi:10.1039/9781782622345-00001. ISBN 978-1-78262-189-8.
- "Vaccine Testing and the Approval Process". US Centers for Disease Control and Prevention. 1 May 2014. Retrieved 21 March 2020.
- Kessler DA, Feiden KL (March 1995). "Faster evaluation of vital drugs". Scientific American. 272 (3): 48–54. Bibcode:1995SciAm.272c..48K. doi:10.1038/scientificamerican0395-48. PMID 7871409.
- "Coronavirus Treatment Acceleration Program (CTAP)". U.S. Food and Drug Administration (FDA). 2020-04-20. Retrieved 2020-04-27.
- Blackwell JR, Martz M. "Richmond startup awarded $354 million federal contract to make ingredients for COVID-19 drugs" (video). Richmond Times-Dispatch. Retrieved 21 May 2020.
- "Vaccine Product Approval Process". U.S. Food and Drug Administration (FDA). 30 January 2018. Retrieved 21 March 2020.
- "About the Innovative Medicines Initiative". European Innovative Medicines Initiative. 2020. Retrieved 24 January 2020.
- "Critical Path Initiative". U.S. Food and Drug Administration (FDA). 23 April 2018. Retrieved 24 January 2020.
- "Breakthrough Therapy". U.S. Food and Drug Administration (FDA). 4 January 2018. Retrieved 24 January 2020.
- "SARS-CoV-2 Diagnostic Pipeline". Foundation for Innovative New Diagnostics. 2020. Retrieved 4 April 2020.
- "CEPI welcomes UK Government's funding and highlights need for $2 billion to develop a vaccine against COVID-19". Coalition for Epidemic Preparedness Innovations, Oslo, Norway. 6 March 2020. Retrieved 23 March 2020.
- Kelland K (10 March 2020). "Epidemic response group ups coronavirus vaccine funding to $23.7 million". Reuters. Retrieved 21 March 2020.
- "Government of Canada funds 49 additional COVID-19 research projects – Details of the funded projects". Government of Canada. 23 March 2020. Retrieved 23 March 2020.
- Abedi M (23 March 2020). "Canada to spend $192M on developing COVID-19 vaccine". Global News. Retrieved 24 March 2020.
- O'Brien C (31 March 2020). "Vaccine watch: These are the efforts being made around the world". CTV News. Retrieved 1 April 2020.
- "Gates Foundation Expands Commitment to COVID-19 Response, Calls for International Collaboration". Bill & Melinda Gates Foundation. 2020-04-15. Retrieved 2020-04-27.
- Shankland S (2020-03-23). "Sixteen supercomputers tackle coronavirus cures in the US". CNET. ViacomCBS. Retrieved 27 April 2020.
- "The COVID-19 High Performance Computing Consortium". The COVID-19 High Performance Computing Consortium. 2020. Retrieved 2020-04-27.
- "C3.ai, Microsoft, and Leading Universities Launch C3.ai Digital Transformation Institute". C3.ai. 2020-03-26. Retrieved 27 April 2020.
- Broad W (26 March 2020). "A.I. Versus the Coronavirus". The New York Times. The New York Times Company. Retrieved 27 April 2020.
- Broekhuijsen N (3 March 2020). "Help Cure Coronavirus with Your PC's Leftover Processing Power". Tom's Hardware. Retrieved 12 March 2020.
- Bowman G (27 February 2020). "Folding@home takes up the fight against COVID-19 / 2019-nCoV". Folding@home. Retrieved 12 March 2020.
- "Folding@home Turns Its Massive Crowdsourced Computer Network Against COVID-19". March 16, 2020.
- "Rosetta@home Rallies a Legion of Computers Against the Coronavirus". HPCwire. 2020-03-24. Retrieved 2020-11-04.
- "OpenPandemics – COVID-19". IBM. 2020. Retrieved 18 May 2020.
- Kaiser AJ (1 April 2018). "FDA update (2018) – The FDA's new drug approval process: Development and premarket applications". Drug Development and Delivery. Retrieved 25 March 2020.
- "Adaptive Designs for Clinical Trials of Drugs and Biologics: Guidance for Industry" (PDF). U.S. Food and Drug Administration (FDA). 1 November 2019. Retrieved 3 April 2020.
- Pallmann P, Bedding AW, Choodari-Oskooei B, Dimairo M, Flight L, Hampson LV, et al. (February 2018). "Adaptive designs in clinical trials: why use them, and how to run and report them". BMC Medicine. 16 (1): 29. doi:10.1186/s12916-018-1017-7. PMC 5830330. PMID 29490655.
- "Launch of a European clinical trial against COVID-19". INSERM. 22 March 2020. Retrieved 5 April 2020.
The great strength of this trial is its "adaptive" nature. This means that ineffective experimental treatments can very quickly be dropped and replaced by other molecules that emerge from research efforts. We will therefore be able to make changes in real time, in line with the most recent scientific data, in order to find the best treatment for our patients
- Kotok A (19 March 2020). "WHO beginning Covid-19 therapy trial". Technology News: Science and Enterprise. Retrieved 7 April 2020.
- Van Norman GA (June 2019). "Phase II Trials in Drug Development and Adaptive Trial Design". JACC. Basic to Translational Science. 4 (3): 428–437. doi:10.1016/j.jacbts.2019.02.005. PMC 6609997. PMID 31312766.
- Sato A, Shimura M, Gosho M (April 2018). "Practical characteristics of adaptive design in Phase 2 and 3 clinical trials". Journal of Clinical Pharmacy and Therapeutics. 43 (2): 170–180. doi:10.1111/jcpt.12617. PMID 28850685. S2CID 3704071.
- Fogel DB (September 2018). "Factors associated with clinical trials that fail and opportunities for improving the likelihood of success: A review". Contemporary Clinical Trials Communications. 11: 156–164. doi:10.1016/j.conctc.2018.08.001. PMC 6092479. PMID 30112460.
- "R&D costs are on the rise". Medical Marketing and Media. 38 (6): 14. June 1, 2003. Archived from the original on October 18, 2016.
- "Clinical development success rates: 2006–2015" (PDF). BIO Industry Analysis. June 2016.
- Wang Y (2012). "Extracting knowledge from failed development programmes". Pharmaceutical Medicine. 26 (2): 91–96. doi:10.1007/BF03256897. S2CID 17171991.
- Paul SM, Mytelka DS, Dunwiddie CT, Persinger CC, Munos BH, Lindborg SR, Schacht AL (March 2010). "How to improve R&D productivity: the pharmaceutical industry's grand challenge". Nature Reviews. Drug Discovery. 9 (3): 203–214. doi:10.1038/nrd3078. PMID 20168317. S2CID 1299234.
- Prasad V, Mailankody S (November 2017). "Research and Development Spending to Bring a Single Cancer Drug to Market and Revenues After Approval". JAMA Internal Medicine. 177 (11): 1569–1575. doi:10.1001/jamainternmed.2017.3601. PMC 5710275. PMID 28892524.
- Moore TJ, Zhang H, Anderson G, Alexander GC (November 2018). "Estimated Costs of Pivotal Trials for Novel Therapeutic Agents Approved by the US Food and Drug Administration, 2015–2016". JAMA Internal Medicine. 178 (11): 1451–1457. doi:10.1001/jamainternmed.2018.3931. PMC 6248200. PMID 30264133.
- DiMasi JA, Grabowski HG, Hansen RW (May 2016). "Innovation in the pharmaceutical industry: New estimates of R&D costs". Journal of Health Economics. 47: 20–33. doi:10.1016/j.jhealeco.2016.01.012. hdl:10161/12742. PMID 26928437.
- Sertkaya A, Wong HH, Jessup A, Beleche T (April 2016). "Key cost drivers of pharmaceutical clinical trials in the United States". Clinical Trials. 13 (2): 117–26. doi:10.1177/1740774515625964. PMID 26908540. S2CID 24308679.
- Herper M (11 August 2013). "The cost of creating a new drug now $5 billion, pushing Big Pharma to change". Forbes. Retrieved 17 July 2016.
- Maxmen A (August 2016). "Busting the billion-dollar myth: how to slash the cost of drug development". Nature. 536 (7617): 388–90. Bibcode:2016Natur.536..388M. doi:10.1038/536388a. PMID 27558048.
- "CEPI's response to COVID-19". Coalition for Epidemic Preparedness Innovation, Oslo, Norway. 1 March 2020. Retrieved 25 March 2020.
- "COVID-19 Therapeutics Accelerator: Bill & Melinda Gates Foundation, Wellcome, and Mastercard Launch Initiative to Speed Development and Access to Therapies for COVID-19". Bill and Melinda Gates Foundation. 10 March 2020. Retrieved 4 April 2020.
- Thorlund K, Dron L, Park J, Hsu G, Forrest JI, Mills EJ (June 2020). "A real-time dashboard of clinical trials for COVID-19". The Lancet. Digital Health. 2 (6): e286–e287. doi:10.1016/S2589-7500(20)30086-8. PMC 7195288. PMID 32363333.
- "What are the phases of clinical trials?". American Cancer Society. 2020. Retrieved 4 April 2020.
- "'Solidarity' clinical trial for COVID-19 treatment". World Health Organization. 2020-04-27. Retrieved 2020-05-01.
- "WHO Director-General's opening remarks at the media briefing on COVID-19". World Health Organization. 2020-05-25. Retrieved 2020-05-27.
- Maria Cheng, Jamey Keaten (2020-05-25). "WHO pauses hydroxychloroquine coronavirus trial over safety concerns". Global News. The Associated Press. Retrieved 2020-05-27.CS1 maint: uses authors parameter (link)
- Abantika G, Mascarenhas A (4 June 2020). "WHO restarts HCQ trial after Lancet concern over paper that trashed it". indianexpress.com. The Indian Express.
When contacted, Soumya Swaminathan, chief scientist at WHO, told The Indian Express: Our data safety monitoring board reviewed the mortality data in Solidarity... They did not have concerns related to mortality between HCQ and standard of care. Hence, we have decided to resume the trial.
- Mulier T (2020-06-17). "Hydroxychloroquine halted in WHO-sponsored COVID-19 trials". Bloomberg. Retrieved 2020-06-17.
- Branswell H (18 March 2020). "WHO to launch multinational trial to jumpstart search for coronavirus drugs". STAT. Retrieved 28 March 2020.
- Mullard A (April 2020). "Flooded by the torrent: the COVID-19 drug pipeline". Lancet. 395 (10232): 1245–1246. doi:10.1016/S0140-6736(20)30894-1. PMC 7162641. PMID 32305088.
- "WHO Director-General's opening remarks at the media briefing on COVID-19". United Nations, World Health Organization. 27 March 2020. Retrieved 28 March 2020.
- "RECOVERY trial rolled out across the UK". Nuffield Department of Population Health. 2020-04-03. Retrieved 2020-04-18.
- Boseley S (2020-04-17). "Coronavirus: world's biggest trial of drug to treat Covid-19 begins in UK". The Guardian. ISSN 0261-3077. Retrieved 2020-04-18.
- "RECOVERY Trial". Nuffield Department of Population Health. 2020-04-03. Retrieved 2020-04-18.
- "No clinical benefit from use of hydroxychloroquine in hospitalised patients with COVID-19". Recovery Trial, Nuffield Department of Population Health, University of Oxford, UK. 5 June 2020. Retrieved 7 June 2020.
- "Low-cost dexamethasone reduces death by up to one third in hospitalised patients with severe respiratory complications of COVID-19" (PDF). 2020-06-16. Retrieved 2020-06-21.
- Roberts M (16 June 2020). "Coronavirus: Dexamethasone proves first life-saving drug". BBC News Online.
- Clinical trial number NCT04280705 for "Adaptive COVID-19 Treatment Trial (ACTT)" at ClinicalTrials.gov
- "Remdesivir approval status". Drugs.com. 24 March 2020. Retrieved 6 April 2020.
- Pagliarulo N (5 March 2020). "A closer look at the Ebola drug that's become the top hope for a coronavirus treatment". BioPharma Dive. Retrieved 19 March 2020.
There's only one drug right now that we think may have real efficacy. And that's remdesivir." said Bruce Aylward, a senior advisor and international leader of the World Health Organization's joint mission to China
- "Emergency access to remdesivir outside of clinical trials". Gilead Sciences. 1 April 2020. Retrieved 7 April 2020.
- "Information for clinicians on therapeutic options for COVID-19 patients". US Centers for Disease Control and Prevention. 21 March 2020. Retrieved 22 March 2020.
- "NIH clinical trial shows remdesivir accelerates recovery from advanced COVID-19" (Press release). US National Institute of Allergy and Infectious Diseases. 2020-04-29. Retrieved 2020-04-29.
- Wang Y, Zhang D, Du G, Du R, Zhao J, Jin Y, et al. (May 2020). "Remdesivir in adults with severe COVID-19: a randomised, double-blind, placebo-controlled, multicentre trial". Lancet. 395 (10236): 1569–1578. doi:10.1016/S0140-6736(20)31022-9. PMC 7190303. PMID 32423584.
- "Hydroxychloroquine sulfate". Drugs.com. 31 March 2020. Retrieved 5 April 2020.
- "Chloroquine phosphate". Drugs.com. 31 March 2020. Retrieved 5 April 2020.
- "Fujifilm Announces the Start of a Phase III Clinical Trial of Influenza Antiviral Drug Avigan (favipiravir) on COVID-19 in Japan and Commits to Increasing Production". Drugs.com via Fujifilm Toyama Chemical Co., Ltd. 31 March 2020. Retrieved 6 April 2020.
- Gregory A (18 March 2020). "Coronavirus: Japanese anti-viral drug effective in treating patients, Chinese official says". The Independent. Retrieved 19 March 2020.
- "Ritonavir". Drugs.com. 2020. Retrieved 6 April 2020.
- "Kevzara". Drugs.com. 7 March 2019. Retrieved 6 April 2020.
- Staines R (31 March 2020). "Sanofi begins trial of Kevzara against COVID-19 complications". PharmaPhorum. Retrieved 6 April 2020.
- McGrath J (2 April 2020). "All the COVID-19 vaccines and treatments currently in clinical trials". Digital Trends. Retrieved 6 April 2020.
- "Tocilizumab". Drugs.com. 7 June 2019. Retrieved 6 April 2020.
- Slater H (26 March 2020). "FDA approves Phase III clinical trial of tocilizumab for COVID-19 pneumonia". Cancer Network, MJH Life Sciences. Retrieved 28 March 2020.
- "Roche provides an update on the phase III COVACTA trial of Actemra/RoActemra in hospitalised patients with severe COVID-19 associated pneumonia". Hoffmann-La Roche. 29 July 2020. Retrieved 2020-08-18.
- Clinical trial number NCT04351152 for "Phase 3 Study to Evaluate Efficacy and Safety of Lenzilumab in Hospitalized Patients With COVID-19 Pneumonia" at ClinicalTrials.gov
- "Dapagliflozin: MedlinePlus Drug Information". medlineplus.gov. 2020-04-20. Retrieved 2020-04-27.
- Clinical trial number NCT04350593 for "Dapagliflozin in Respiratory Failure in Patients With COVID-19 (DARE-19)" at ClinicalTrials.gov
- Clinical trial number NCT04317040 for "CD24Fc as a Non-antiviral Immunomodulator in COVID-19 Treatment (SAC-COVID)" at ClinicalTrials.gov
- Hinton DM (28 March 2020). "Request for Emergency Use Authorization For Use of Chloroquine Phosphate or Hydroxychloroquine Sulfate Supplied From the Strategic National Stockpile for Treatment of 2019 Coronavirus Disease" (PDF). U.S. Food and Drug Administration (FDA). Retrieved 30 March 2020.
- "Fact Sheet for Patients and Parent/Caregivers Emergency Use Authorization (EUA) of Chloroquine Phosphate for Treatment of COVID-19 in Certain Hospitalized Patients" (PDF). FDA.
- Lovelace Jr B (15 June 2020). "FDA revokes emergency use of hydroxychloroquine". CNBC.
- "Revised advisory on the use of Hydroxychloroquine(HCQ) as prophylaxis for SARS-CoV-2 infection(in supersession of previous advisory dated 23rd March, 2020)" (PDF). icmr.gov.in. Indian Council of Medical Research. 22 May 2020. Archived (PDF) from the original on 23 May 2020. Retrieved 3 July 2020.
- Goodman J, Giles C (1 Jul 2020). "Coronavirus and hydroxychloroquine: What do we know?". bbc.com. BBC. Archived from the original on 3 July 2020. Retrieved 3 July 2020.
- "Hydroxychloroquine does not benefit adults hospitalized with COVID-19". National Institutes of Health (NIH) (Press release). 9 November 2020. Retrieved 9 November 2020.
This article incorporates text from this source, which is in the public domain.
- Self WH, Semler MW, Leither LM, Casey JD, Angus DC, Brower RG, et al. (December 2020). "Effect of Hydroxychloroquine on Clinical Status at 14 Days in Hospitalized Patients With COVID-19: A Randomized Clinical Trial". JAMA. 324 (21): 2165–2176. doi:10.1001/jama.2020.22240. PMC 7653542. PMID 33165621.
- Sung-sun K (2020-02-13). "Physicians work out treatment guidelines for coronavirus". Korea Biomedical Review. Retrieved 2020-03-18.
- "Plaquenil (hydroxychloroquine sulfate) dose, indications, adverse effects, interactions... from PDR.net". Physicians' Desk Reference. Retrieved 2020-03-19.
- Cortegiani A, Ingoglia G, Ippolito M, Giarratano A, Einav S (March 2020). "A systematic review on the efficacy and safety of chloroquine for the treatment of COVID-19". Journal of Critical Care. 57: 279–283. doi:10.1016/j.jcrc.2020.03.005. PMC 7270792. PMID 32173110.
- Yao X, Ye F, Zhang M, Cui C, Huang B, Niu P, et al. (March 2020). "In Vitro Antiviral Activity and Projection of Optimized Dosing Design of Hydroxychloroquine for the Treatment of Severe Acute Respiratory Syndrome Coronavirus 2 (SARS-CoV-2)". Clinical Infectious Diseases. 71 (15): 732–739. doi:10.1093/cid/ciaa237. PMC 7108130. PMID 32150618.
- "What are the prospects for a COVID-19 treatment?". The Guardian. 19 March 2020.
- Palca J (April 21, 2020). "NIH Panel Recommends Against Drug Combination Promoted By Trump For COVID-19". NPR.
- "Japanese flu drug 'clearly effective' in treating coronavirus, says China". 18 March 2020.
- "Coronavirus: Japanese anti-viral drug effective in treating patients, Chinese official says". The Independent.
- "Which Covid-19 drugs work best?". MIT Technology Review.
- "Coronavirus, il Veneto sperimenta l'antivirale giapponese Favipiravir. Ma l'Aifa: "Ci sono scarse evidenze scientifiche su efficacia"". Il Fatto Quotidiano (in Italian). 2020-03-22. Retrieved 2020-03-23.
- "AIFA precisa, uso favipiravir per COVID-19 non autorizzato in Europa e USA, scarse evidenze scientifiche sull'efficacia". aifa.gov.it (in Italian). Retrieved 2020-03-23.
- "Russian Ministry of Health approves the first COVID-19 drug Avifavir produced by JV of RDIF and ChemRar". RDIF. 30 May 2020. Retrieved 31 May 2020.
- "Russian Health Ministry approves anti-coronavirus drug Avifavir". BNN Bloomberg. 31 May 2020. Retrieved 31 May 2020.
- "Russia plans coronavirus vaccine clinical trials in two weeks". Reuters. 30 May 2020. Retrieved 31 May 2020.
- "Glenmark's FabiFlu approved for coronavirus treatment in India, costs Rs 103 per tablet". India Today. June 20, 2020. Retrieved 30 June 2020.
- Warren TK, Jordan R, Lo MK, Ray AS, Mackman RL, Soloveva V, et al. (March 2016). "Therapeutic efficacy of the small molecule GS-5734 against Ebola virus in rhesus monkeys". Nature. 531 (7594): 381–385. Bibcode:2016Natur.531..381W. doi:10.1038/nature17180. PMC 5551389. PMID 26934220.
- Lo MK, Jordan R, Arvey A, Sudhamsu J, Shrivastava-Ranjan P, Hotard AL, et al. (March 2017). "GS-5734 and its parent nucleoside analog inhibit filo-, pneumo-, and paramyxoviruses". Scientific Reports. 7: 43395. Bibcode:2017NatSR...743395L. doi:10.1038/srep43395. PMC 5338263. PMID 28262699.
- World Health Organization (2020). Therapeutics and COVID-19: living guideline, 20 November 2020. World Health Organization (WHO) (Report). hdl:10665/336729. WHO/2019-nCov/remdesivir/2020.1.
- Lamontagne F, Agoritsas T, Macdonald H, Leo YS, Diaz J, Agarwal A, et al. (September 2020). "A living WHO guideline on drugs for covid-19". BMJ. 370: m3379. doi:10.1136/bmj.m3379. PMID 32887691. Lay summary.
- Mehta N, Mazer-Amirshahi M, Alkindi N (April 2020). "Pharmacotherapy in COVID-19; A narrative review for emergency providers". The American Journal of Emergency Medicine. 38 (7): S0735-6757(20)30263-1. doi:10.1016/j.ajem.2020.04.035. PMC 7158837. PMID 32336586.
- "Frequently Asked Questions on the Emergency Use Authorization for Remdesivir for Certain Hospitalized COVID‐19 Patients" (PDF). U.S. Food and Drug Administration (FDA). 1 May 2020. Retrieved 1 May 2020.
This article incorporates text from this source, which is in the public domain.
- "Coronavirus (COVID-19) Update: FDA Authorizes Monoclonal Antibodies for Treatment of COVID-19". U.S. Food and Drug Administration (FDA) (Press release). 21 November 2020. Retrieved 21 November 2020.
This article incorporates text from this source, which is in the public domain.
- "EMA starts rolling review of REGN‑COV2 antibody combination (casirivimab / imdevimab)". European Medicines Agency (EMA). 1 February 2021. Retrieved 1 February 2021. Text was copied from this source which is © European Medicines Agency. Reproduction is authorized provided the source is acknowledged.
- "Repurposing drugs". National Center for Advancing Translational Sciences (NCATS). 7 November 2017. Retrieved 26 March 2020.
- Li G, De Clercq E (March 2020). "Therapeutic options for the 2019 novel coronavirus (2019-nCoV)". Nature Reviews. Drug Discovery. 19 (3): 149–150. doi:10.1038/d41573-020-00016-0. PMID 32127666.
- Kruse RL (31 January 2020). "Therapeutic strategies in an outbreak scenario to treat the novel coronavirus originating in Wuhan, China". F1000Research. 9: 72. doi:10.12688/f1000research.22211.1. PMC 7029759. PMID 32117569.
- Mitjà O, Clotet B (March 2020). "Use of antiviral drugs to reduce COVID-19 transmission". The Lancet. Global Health. Elsevier BV. 8 (5): e639–e640. doi:10.1016/s2214-109x(20)30114-5. PMC 7104000. PMID 32199468.
- "UN health chief announces global 'solidarity trial' to jumpstart search for COVID-19 treatment". United Nations – News. World Health Organization. 18 March 2020. Retrieved 29 March 2020.
- Kupferschmidt K, Cohen J (March 2020). "Race to find COVID-19 treatments accelerates". Science. 367 (6485): 1412–1413. Bibcode:2020Sci...367.1412K. doi:10.1126/science.367.6485.1412. PMID 32217705.
- "COVID-19 drug development: Landscape analysis of therapeutics (table)" (PDF). United Nations, World Health Organization. 21 March 2020. Retrieved 29 March 2020.
- "The drug development process: Clinical research". U.S. Food and Drug Administration (FDA). 4 January 2018. Retrieved 28 April 2020.
- Riley Griffin (13 October 2020). "Eli Lilly Covid Antibody Trial Paused Due to Safety Concerns". Bloomberg. Retrieved 13 October 2020.
- Katherine J. Wu, Katie Thomas (13 October 2020). "Eli Lilly's Antibody Trial Is Paused Over Potential Safety Concern". The New York Times. Retrieved 26 October 2020.CS1 maint: uses authors parameter (link)
- "Lilly Statement on the NIAID Decision to Pause Enrollment in ACTIV-3 Clinical Trial". Eli Lilly and Company. 14 October 2020. Retrieved 26 October 2020.
- "Lilly Statement Regarding NIH's ACTIV-3 Clinical Trial". Eli Lilly and Company (Press release). 26 October 2020. Retrieved 26 October 2020.
- "Lilly provides comprehensive update on progress of SARS-CoV-2 neutralizing antibody programs". Eli Lilly and Company. 7 October 2020. Retrieved 26 October 2020.
- "Coronavirus (COVID-19) Update: FDA Authorizes Monoclonal Antibody for Treatment of COVID-19". U.S. Food and Drug Administration (FDA) (Press release). 9 November 2020. Retrieved 9 November 2020.
This article incorporates text from this source, which is in the public domain.
- Zhang L, Lin D, Sun X, Curth U, Drosten C, Sauerhering L, et al. (March 2020). "Crystal structure of SARS-CoV-2 main protease provides a basis for design of improved α-ketoamide inhibitors". Science. 368 (6489): 409–412. Bibcode:2020Sci...368..409Z. doi:10.1126/science.abb3405. PMC 7164518. PMID 32198291.
- Cao B, Wang Y, Wen D, Liu W, Wang J, Fan G, et al. (March 2020). "A Trial of Lopinavir-Ritonavir in Adults Hospitalized with Severe Covid-19". The New England Journal of Medicine. 382 (19): 1787–1799. doi:10.1056/nejmoa2001282. PMC 7121492. PMID 32187464.
- Morse JS, Lalonde T, Xu S, Liu WR (March 2020). "Learning from the Past: Possible Urgent Prevention and Treatment Options for Severe Acute Respiratory Infections Caused by 2019-nCoV". ChemBioChem. 21 (5): 730–738. doi:10.1002/cbic.202000047. PMC 7162020. PMID 32022370.
- Liu C, Zhou Q, Li Y, Garner LV, Watkins SP, Carter LJ, et al. (March 2020). "Research and Development on Therapeutic Agents and Vaccines for COVID-19 and Related Human Coronavirus Diseases". ACS Central Science. 6 (3): 315–331. doi:10.1021/acscentsci.0c00272. PMC 7094090. PMID 32226821.
- Ramajayam R, Tan KP, Liang PH (October 2011). "Recent development of 3C and 3CL protease inhibitors for anti-coronavirus and anti-picornavirus drug discovery". Biochemical Society Transactions. 39 (5): 1371–1375. doi:10.1042/BST0391371. PMID 21936817.
- "Step 2: Preclinical Research". U.S. Food and Drug Administration (FDA). 4 January 2018. Retrieved 23 March 2020.
- Grenfell R, Drew T (14 February 2020). "Here's why the WHO says a coronavirus vaccine is 18 months away". The Conversation. Retrieved 11 November 2020.
- Preston E (19 March 2020). "Why will a coronavirus vaccine take so long?". The Boston Globe. Retrieved 21 March 2020.
- Gates B (February 2020). "Responding to Covid-19 – A Once-in-a-Century Pandemic?". The New England Journal of Medicine. 382 (18): 1677–1679. doi:10.1056/nejmp2003762. PMID 32109012.
- Firth A, Prathapan P (2021-01-01). "Broad-spectrum therapeutics: A new antimicrobial class". Current Research in Pharmacology and Drug Discovery. 2: 100011. doi:10.1016/j.crphar.2020.100011.
- Firth A, Prathapan P (December 2020). "Azithromycin: The First Broad-spectrum Therapeutic". European Journal of Medicinal Chemistry. 207: 112739. doi:10.1016/j.ejmech.2020.112739. PMC 7434625. PMID 32871342.
- "Biodefense Strategic Plan | NIH: National Institute of Allergy and Infectious Diseases". www.niaid.nih.gov. Retrieved 2021-02-02.
- "NIH funds development of new broad-spectrum therapeutics". National Institutes of Health (NIH). 2015-09-18. Retrieved 2021-02-02.
Further reading
- McCreary EK, Pogue JM (April 2020). "Coronavirus Disease 2019 Treatment: A Review of Early and Emerging Options". Open Forum Infectious Diseases. 7 (4): ofaa105. doi:10.1093/ofid/ofaa105. PMC 7144823. PMID 32284951.
External links
- R&D Blueprint and COVID-19, World Health Organization
- Coronaviruses by US National Institute for Allergy and Infectious Diseases
- COVID-19 therapeutics tracker Regulatory Affairs Professionals Society